Abstract
The general formalism required to treat two-state sliding filament models of muscle contraction, including free energy considerations, is first reviewed and amplified. This formalism is then used to examine, and modify as needed, three models studied previously by Podolsky and Nolan, in which cross-bridge attachment-detachment and ATP turnover are not tightly coupled. No attempt is made here to establish an optimal, self-consistent model of this type because our interest is primarily in methadology rather than in fitting experimental results. But it appears from this preliminary study that such a model, with satisfactory mechanical and thermodynamic properties, could be found. An extremely simple but unrealistic two-state model is also studied which is of interest because it demonstrates the fact that it is possible, in principle at least, for sliding filament models to work with very high thermodynamic efficiencies (50-100 percent). An appendix is included that is concerned with the form of the dependence of certain first-order rate constants on the concentrations of ATP, ADP, and P.
Full text
PDF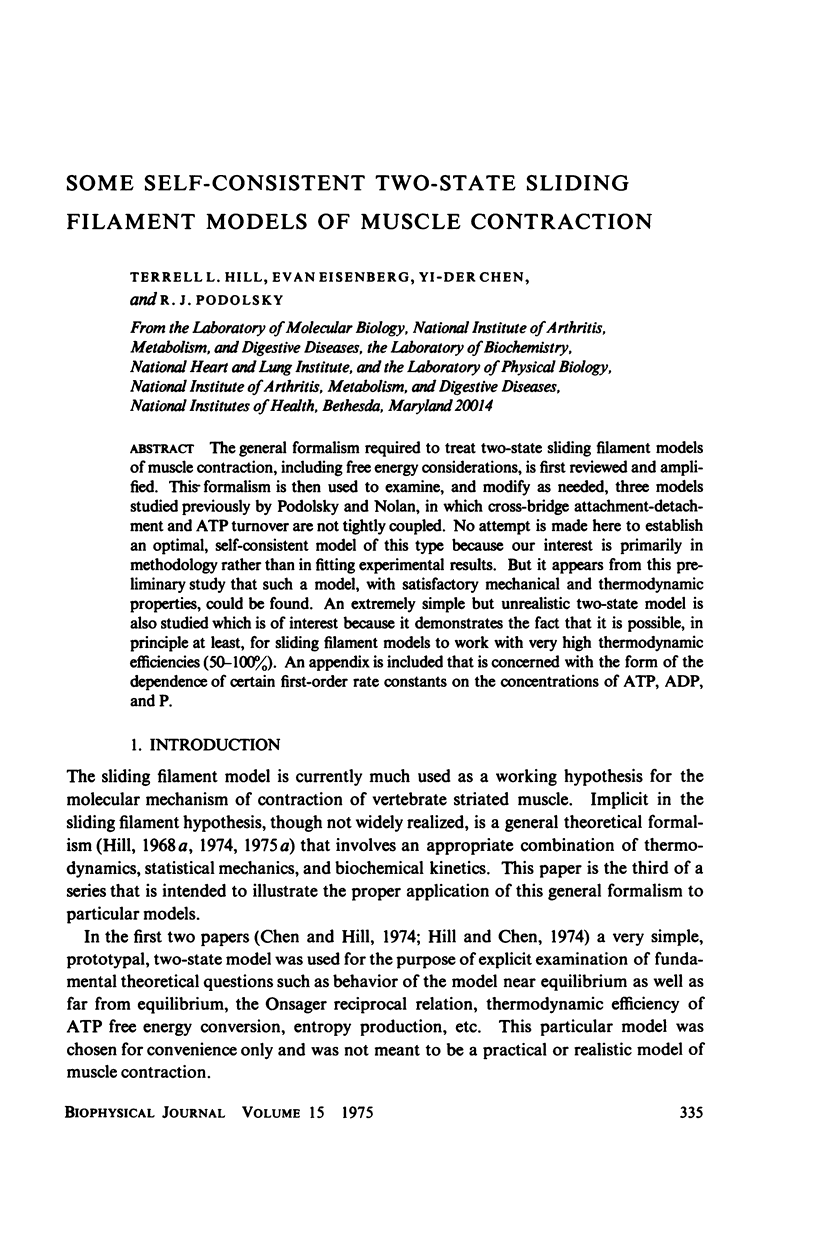
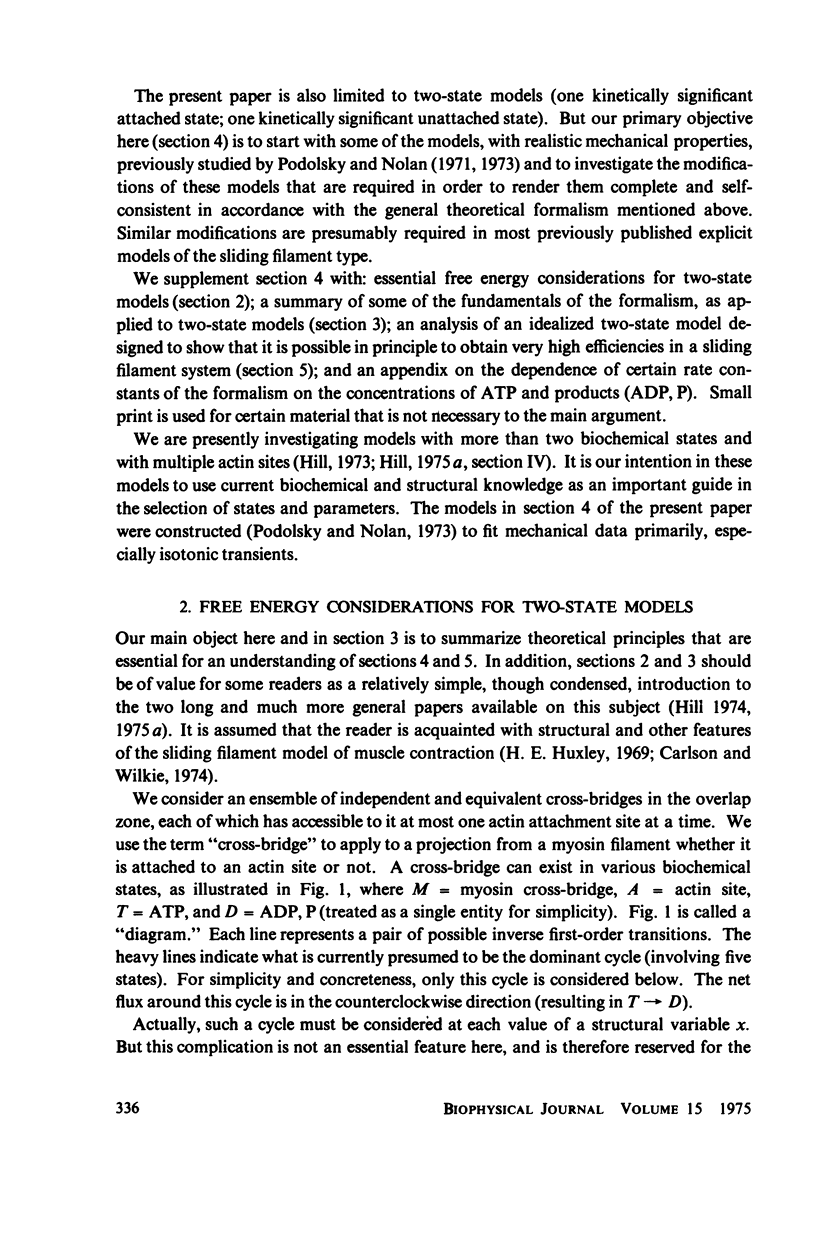
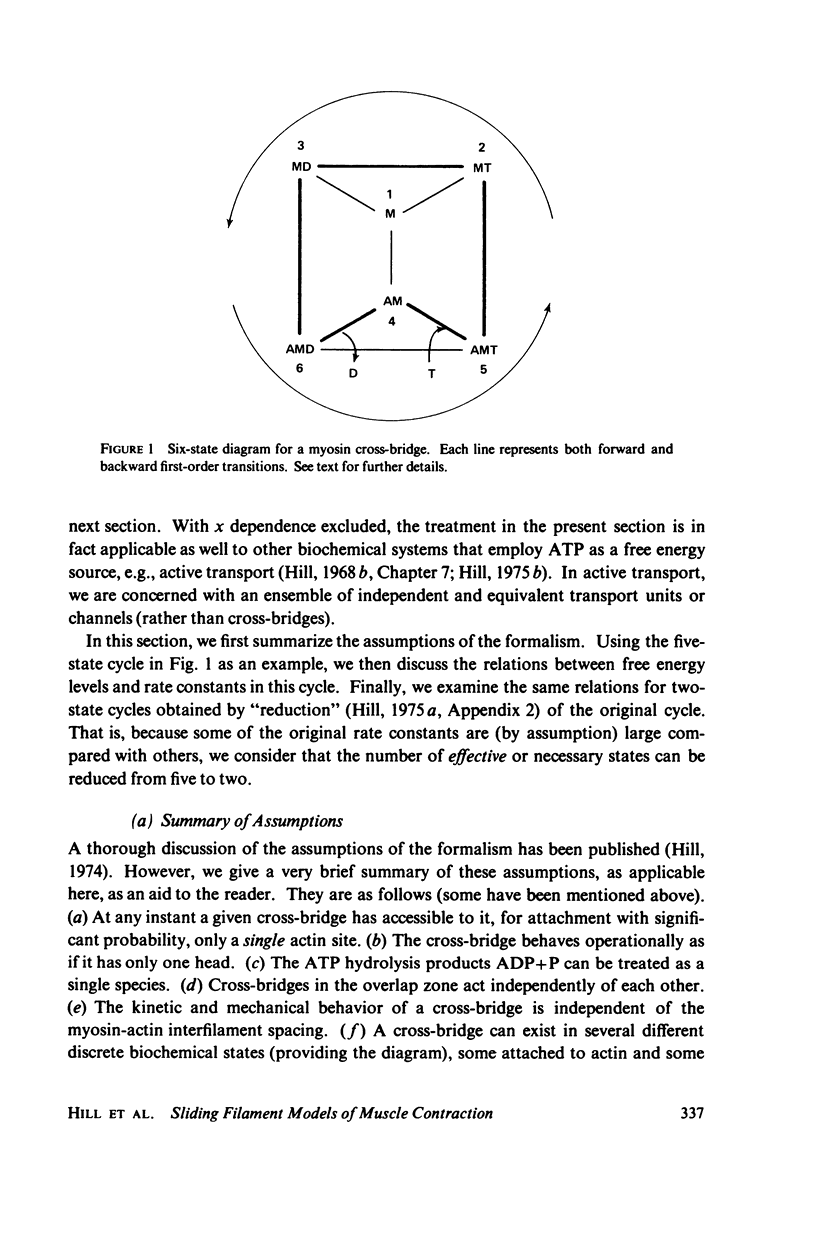
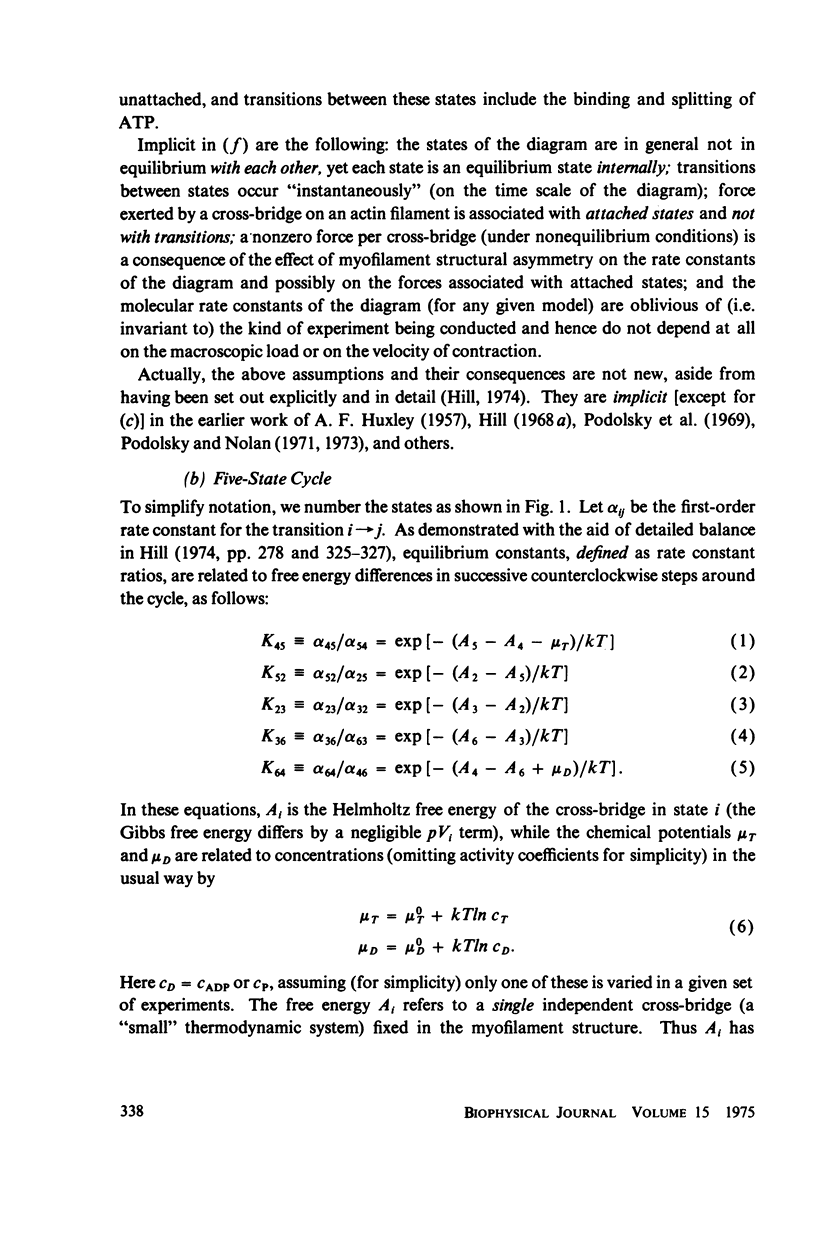
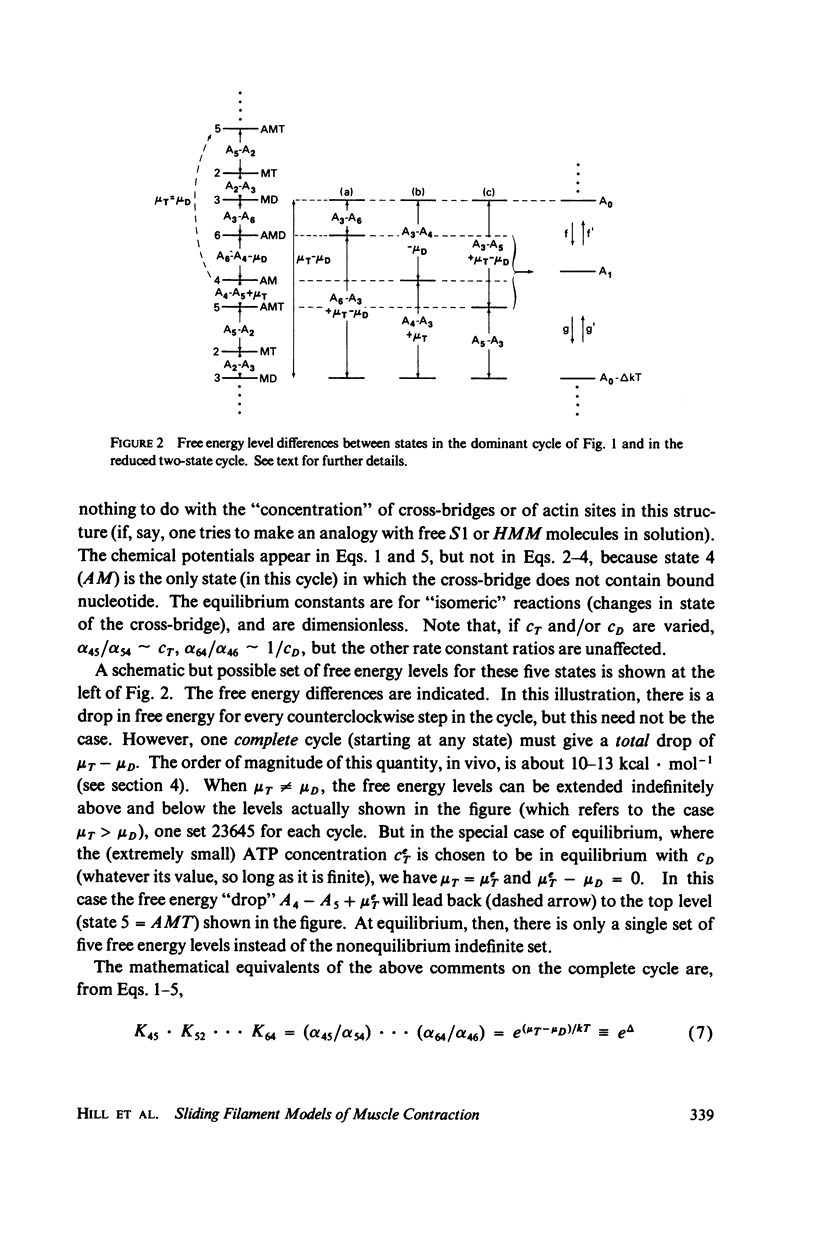
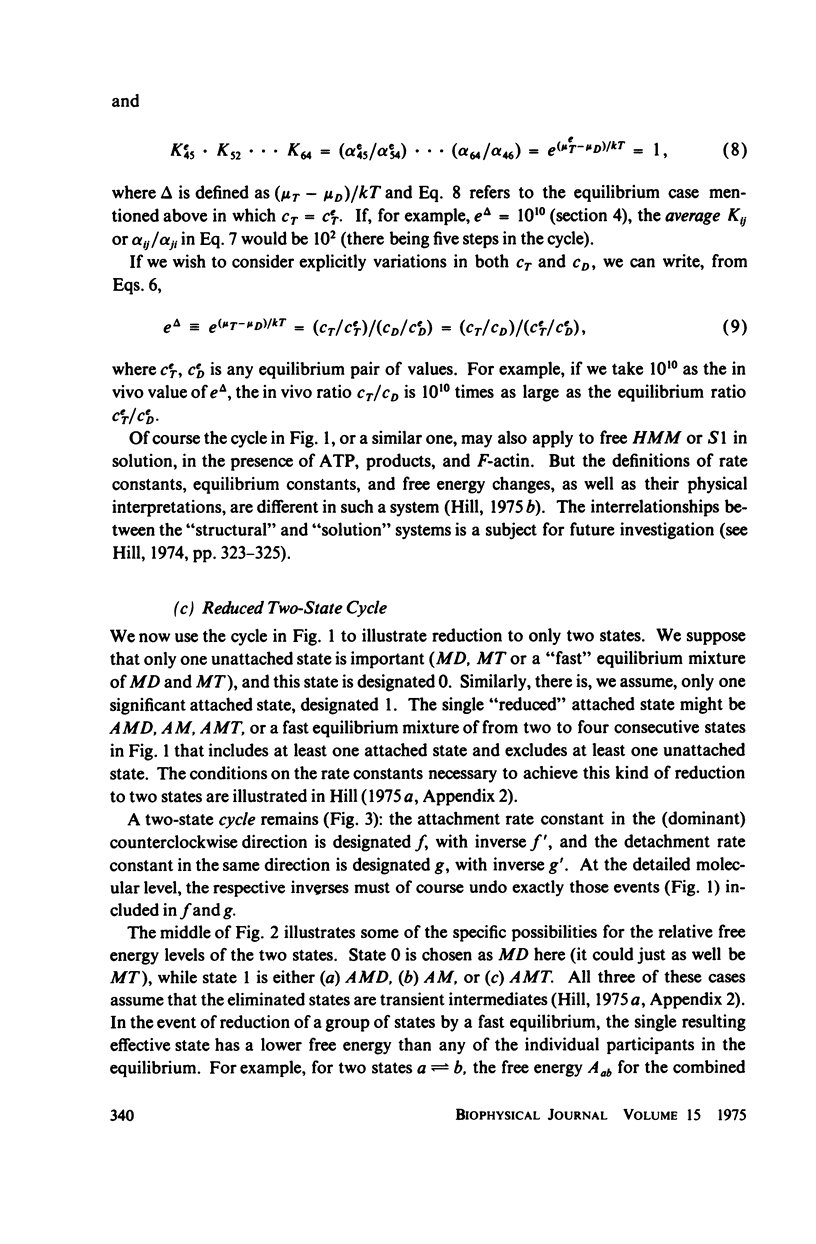
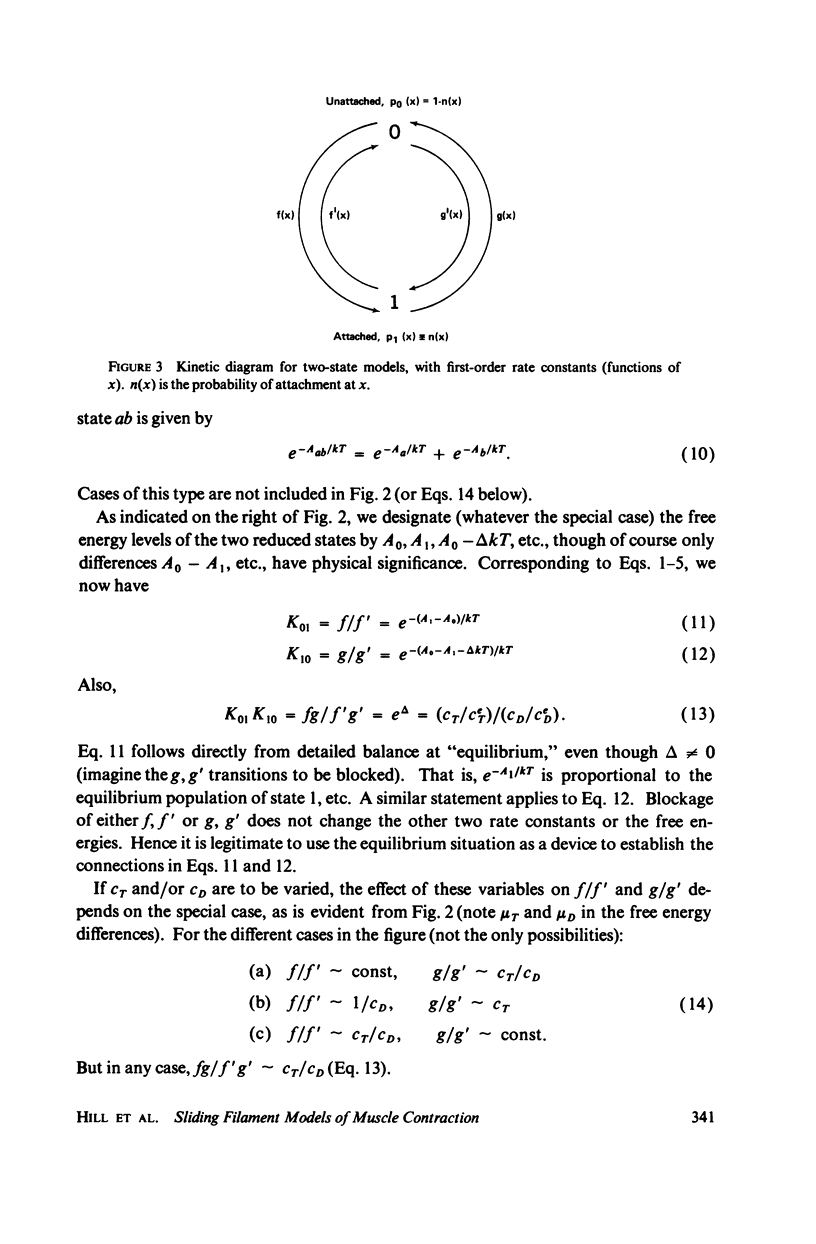
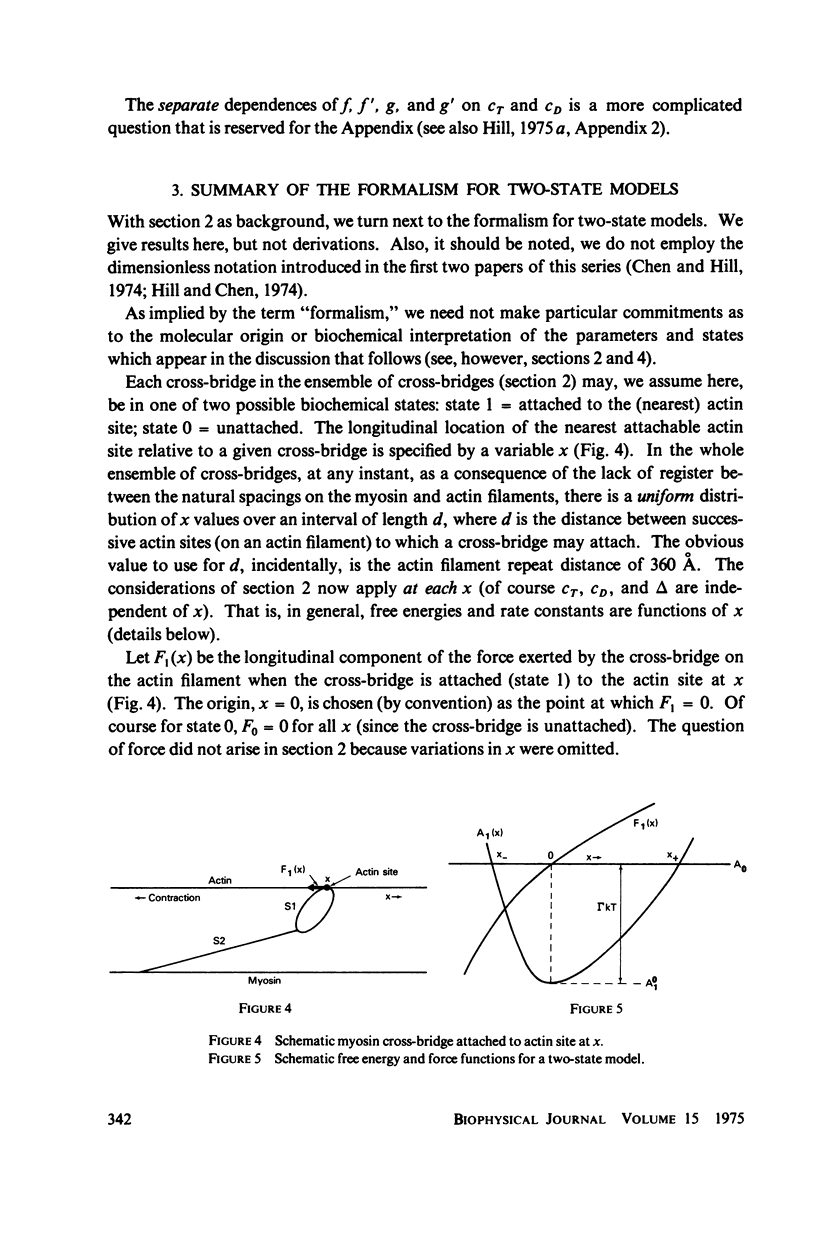
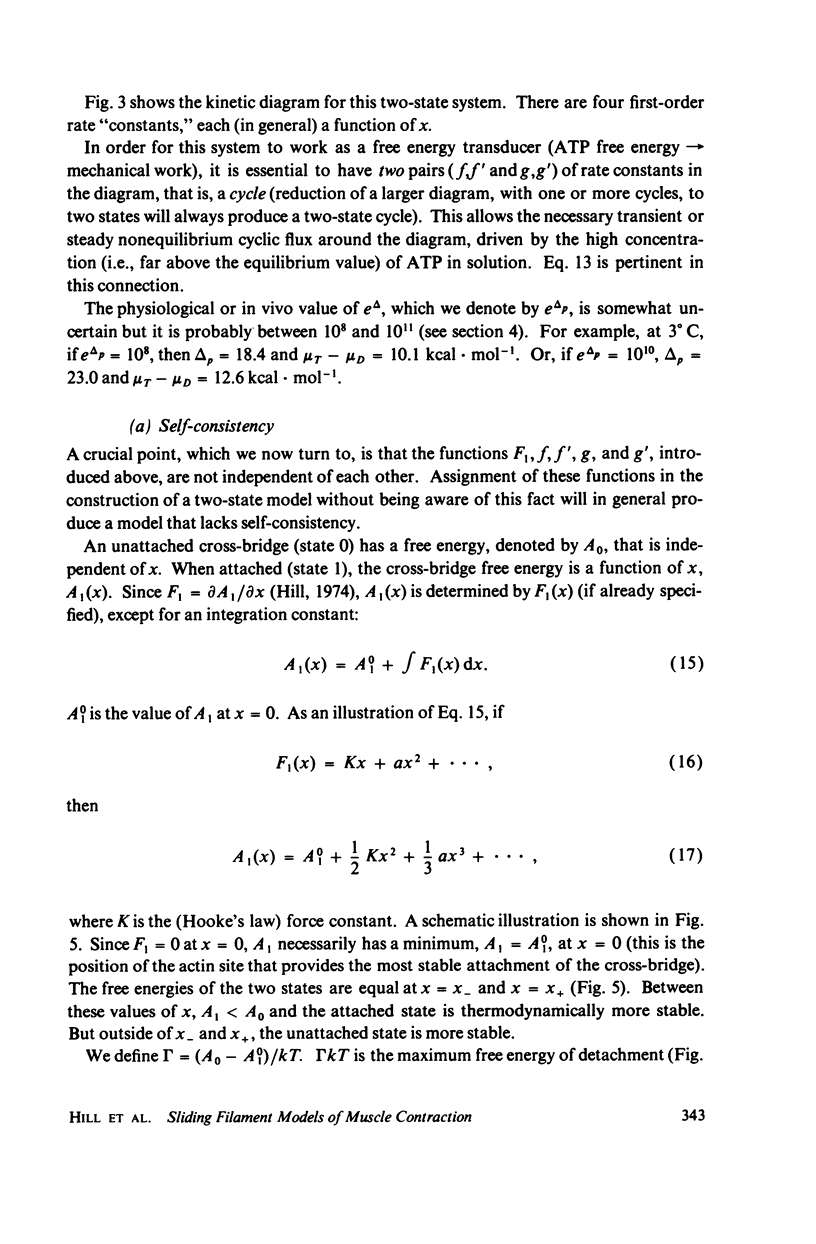
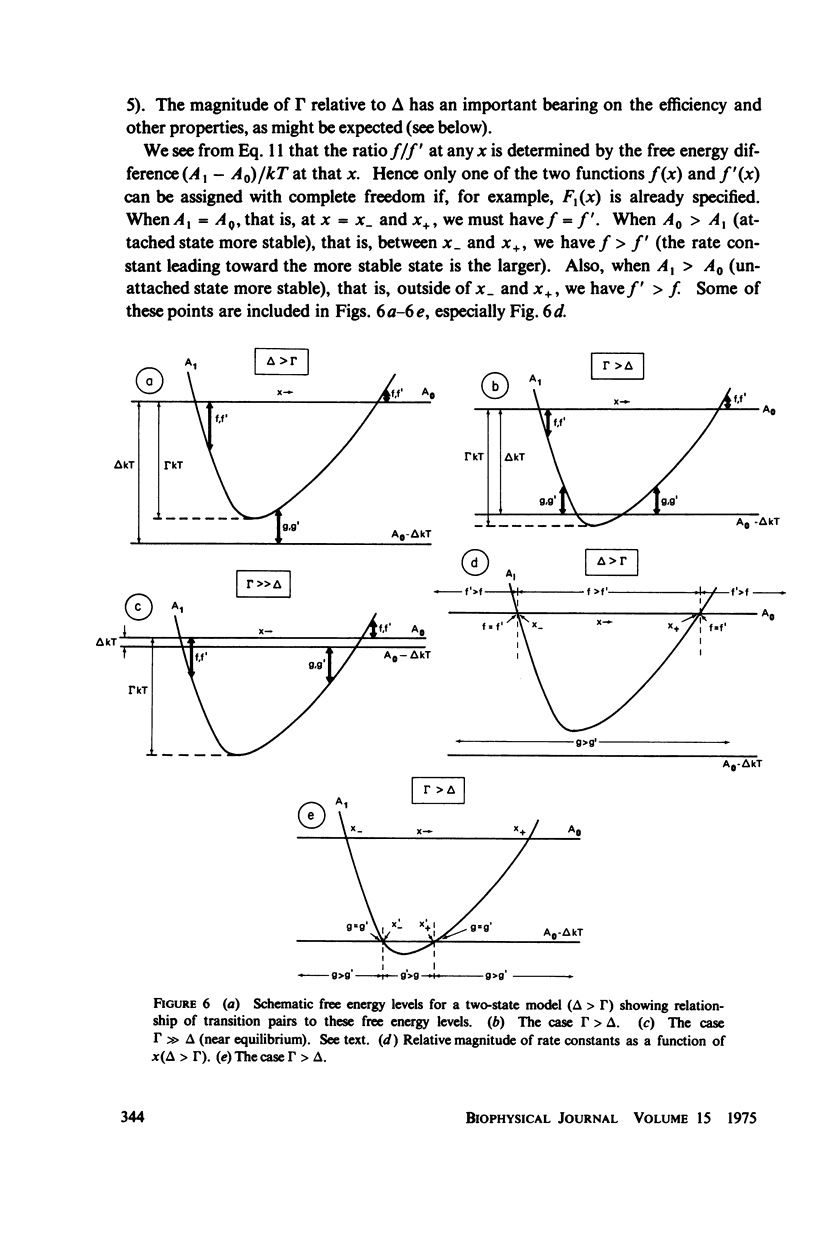
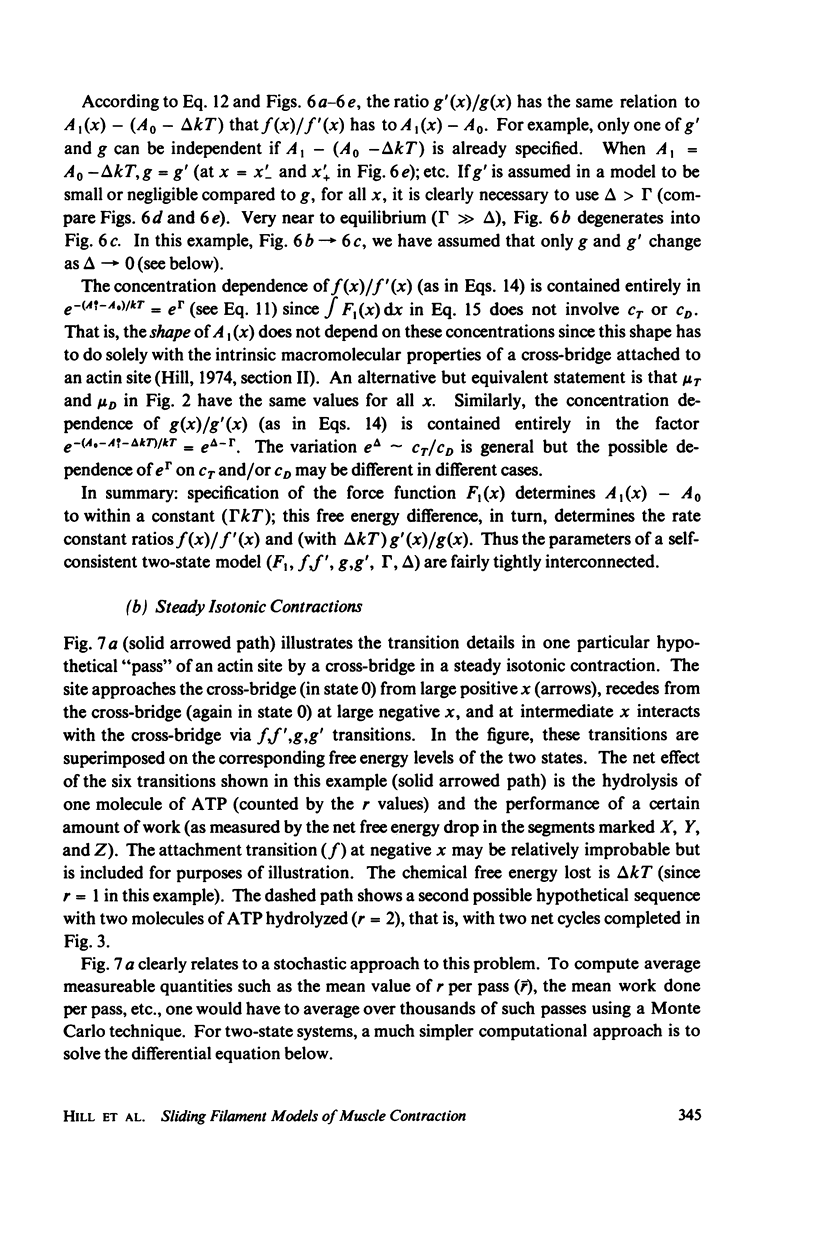
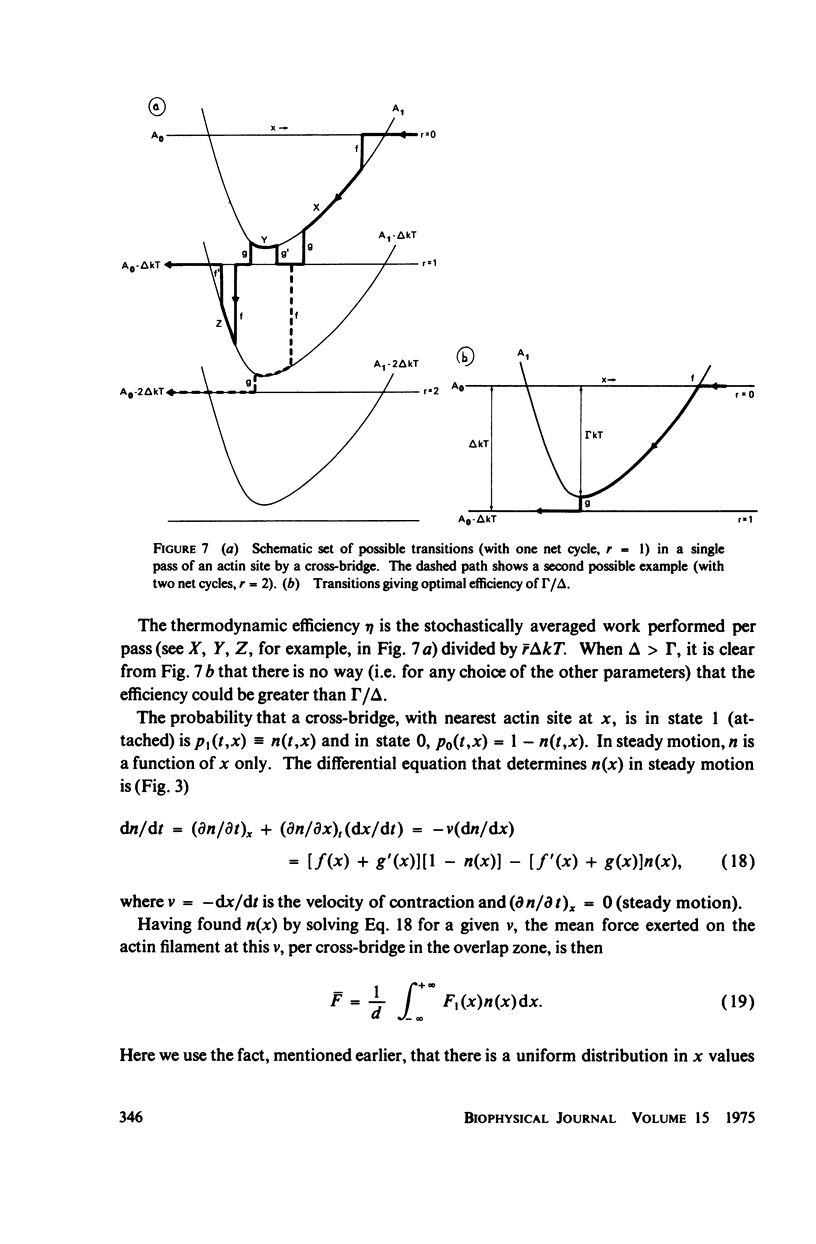
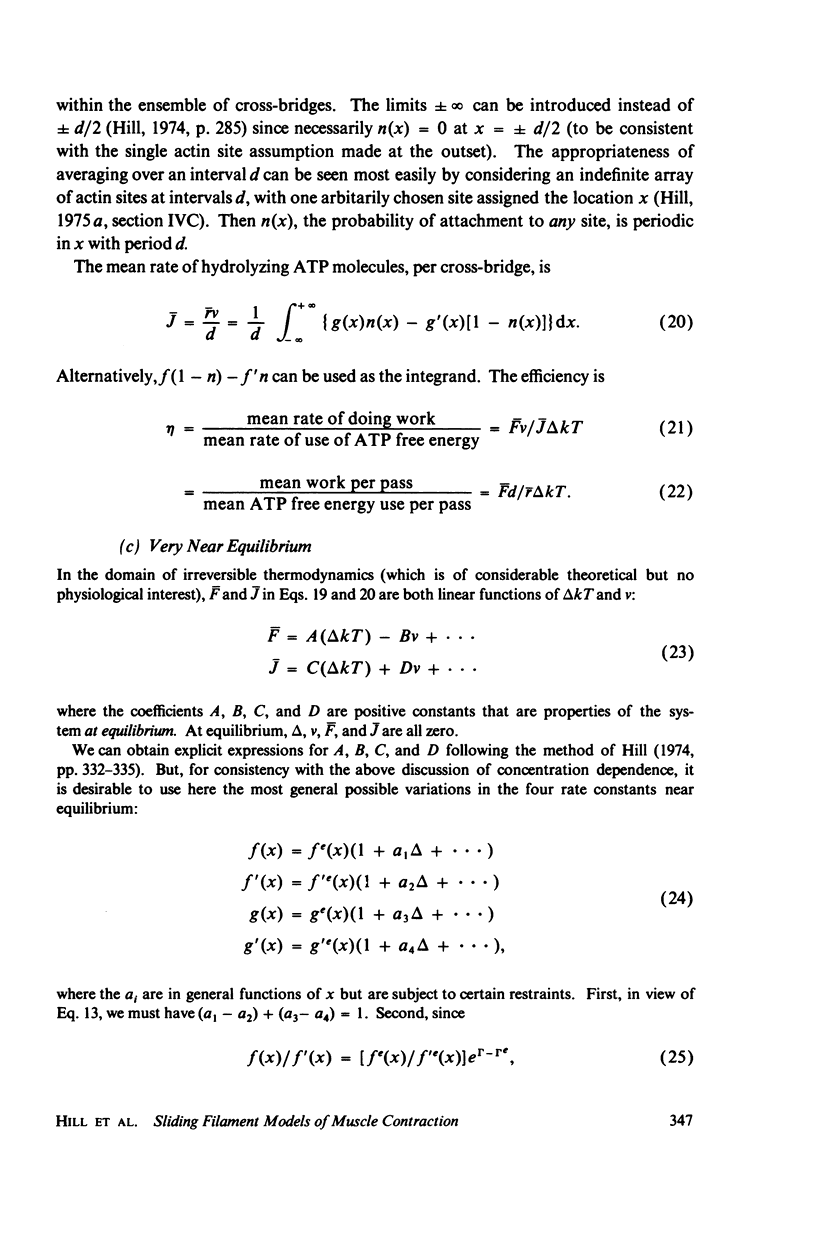
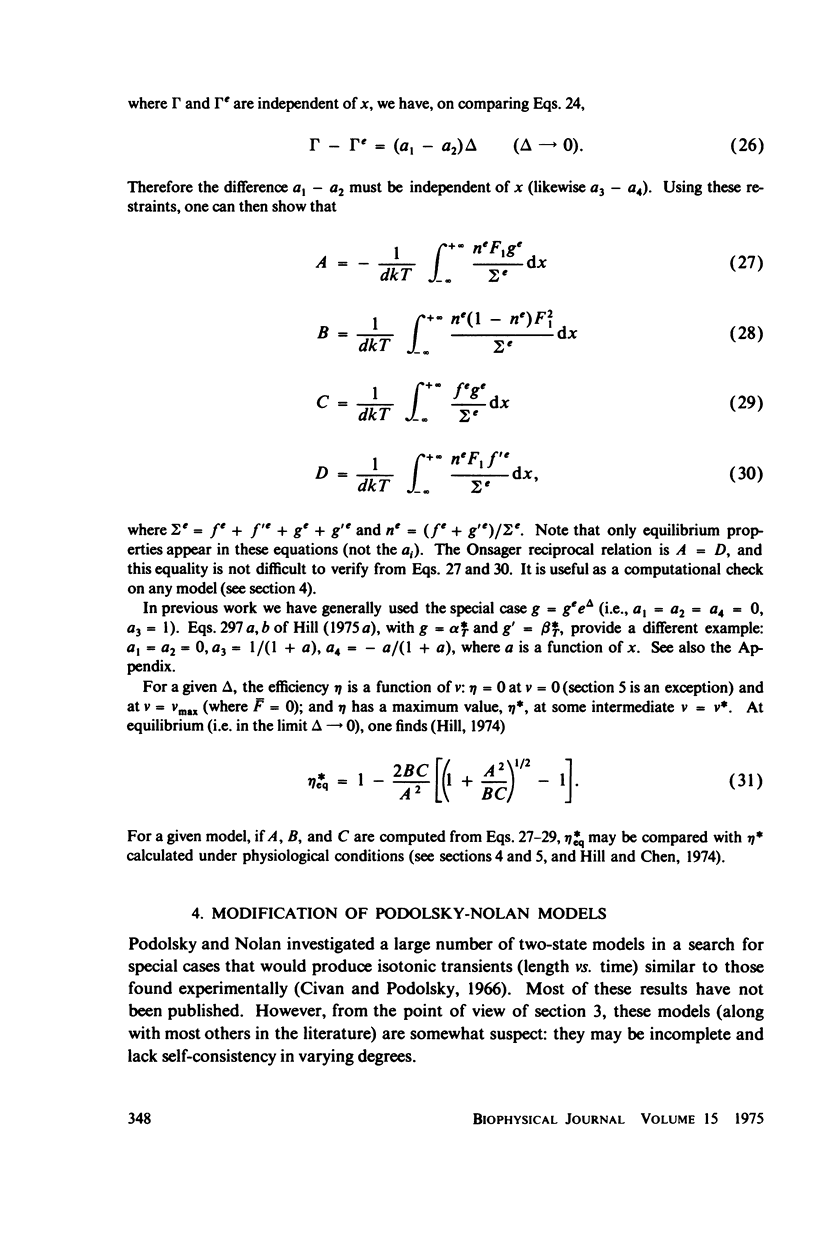
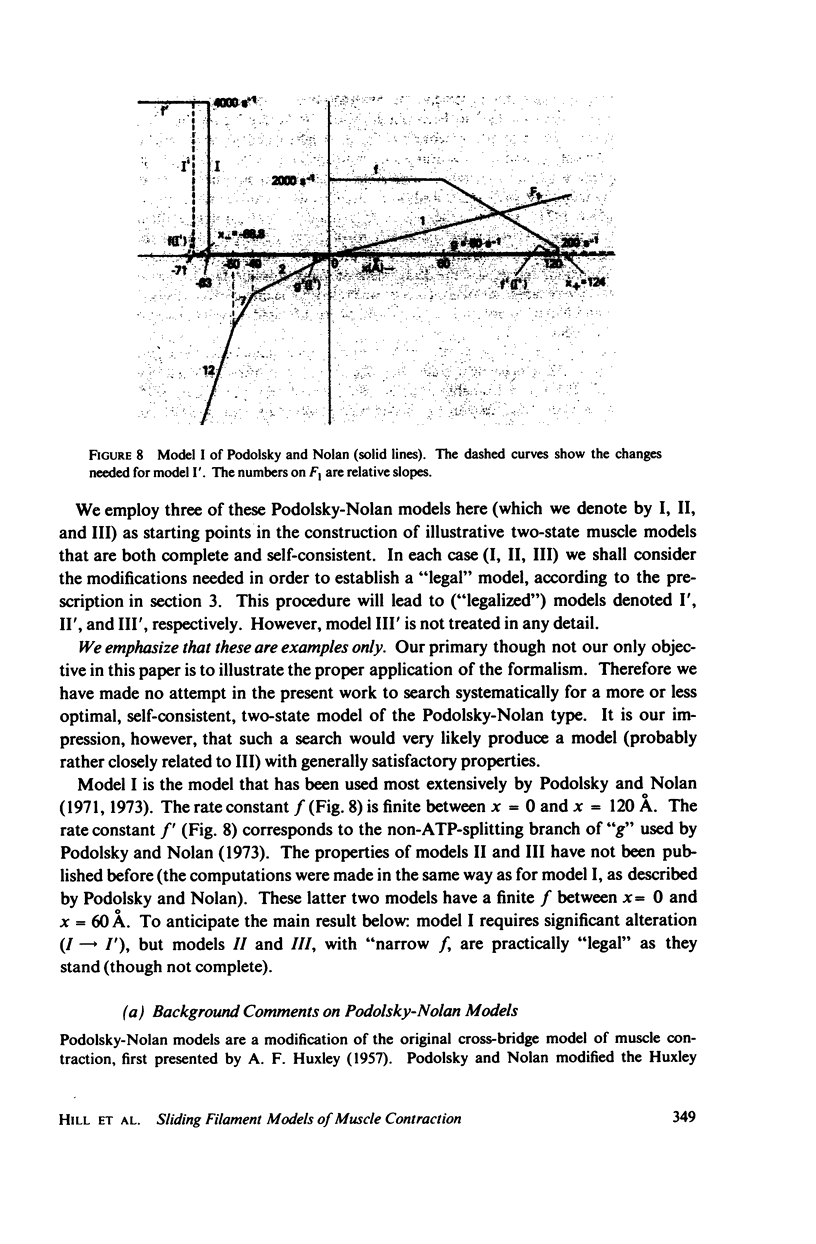
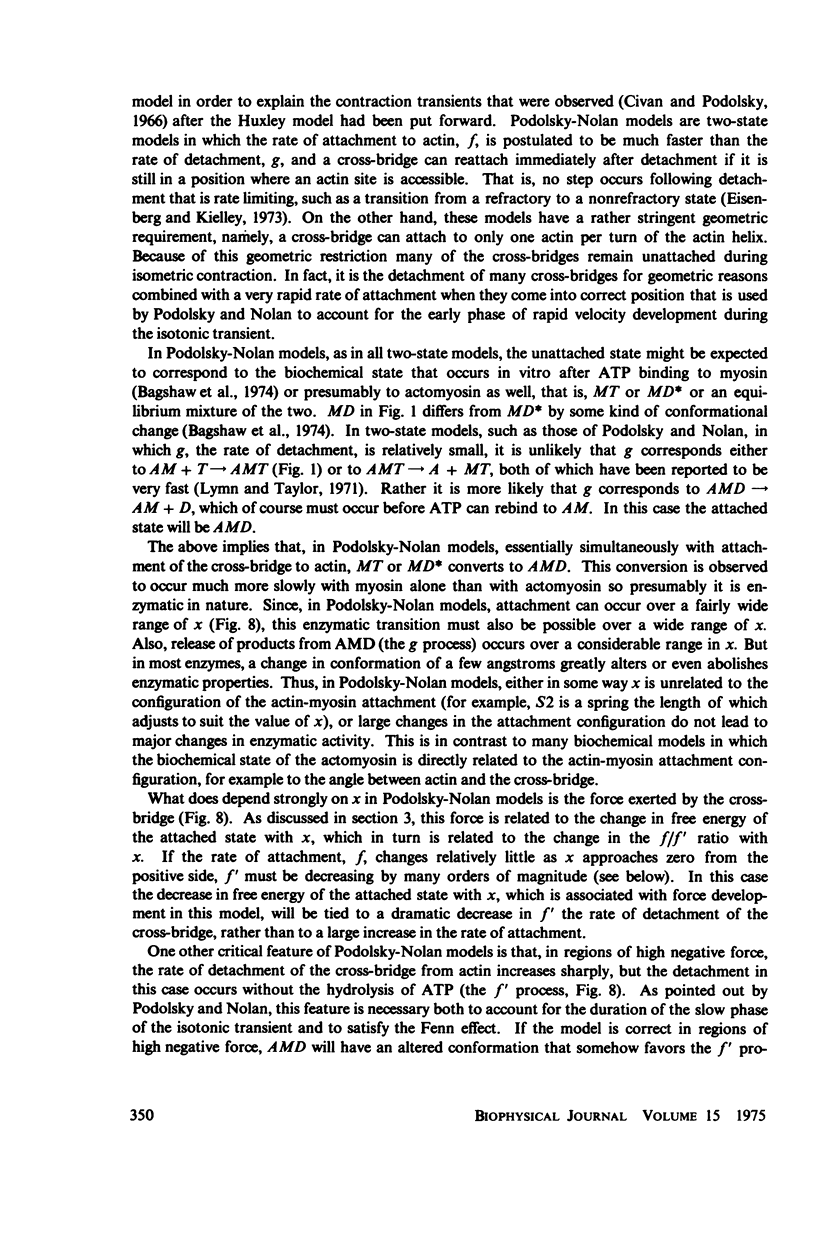
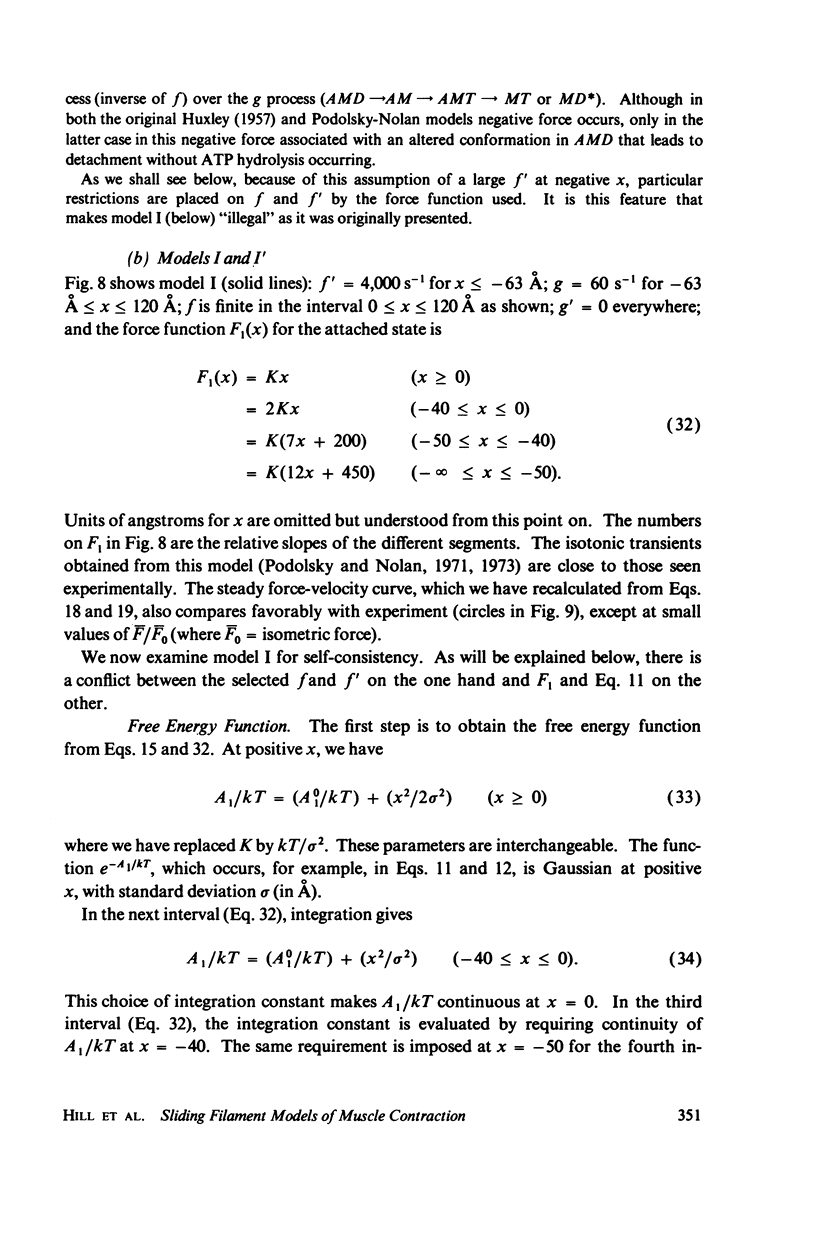
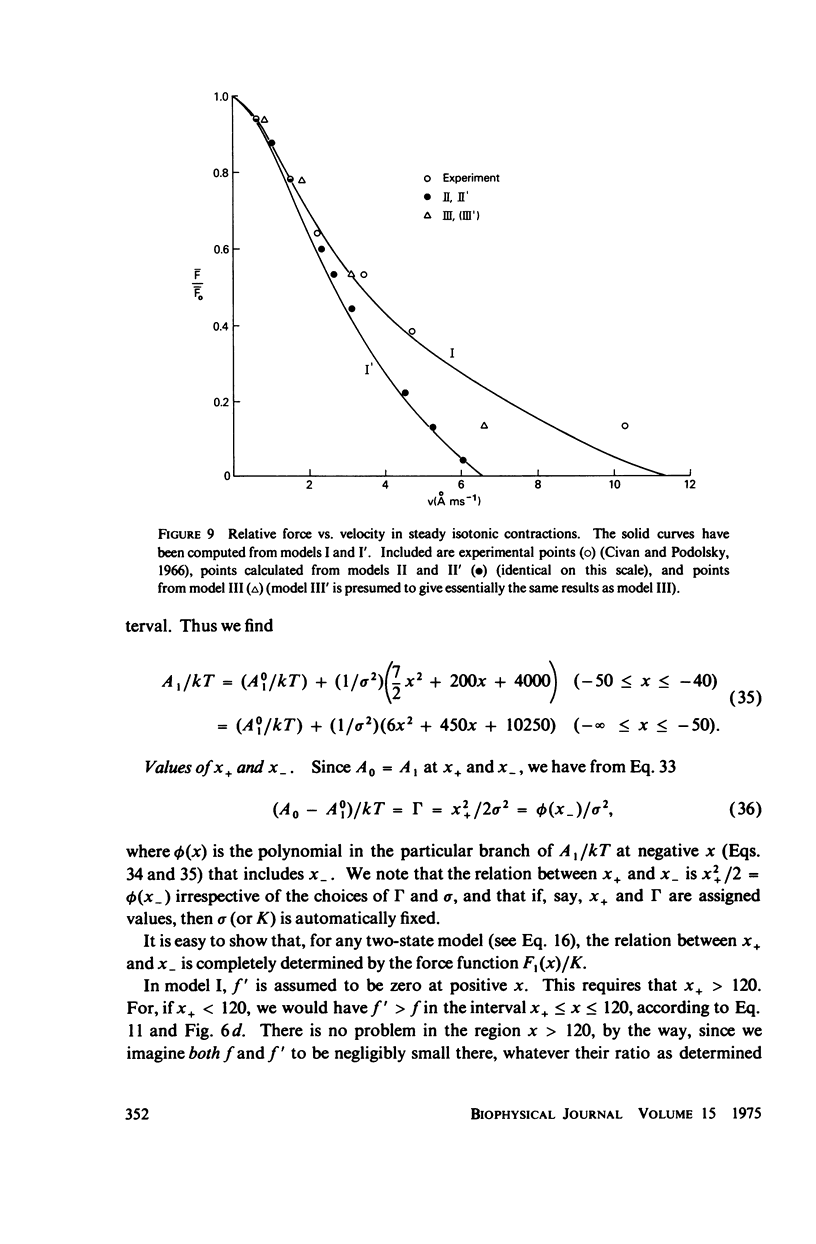
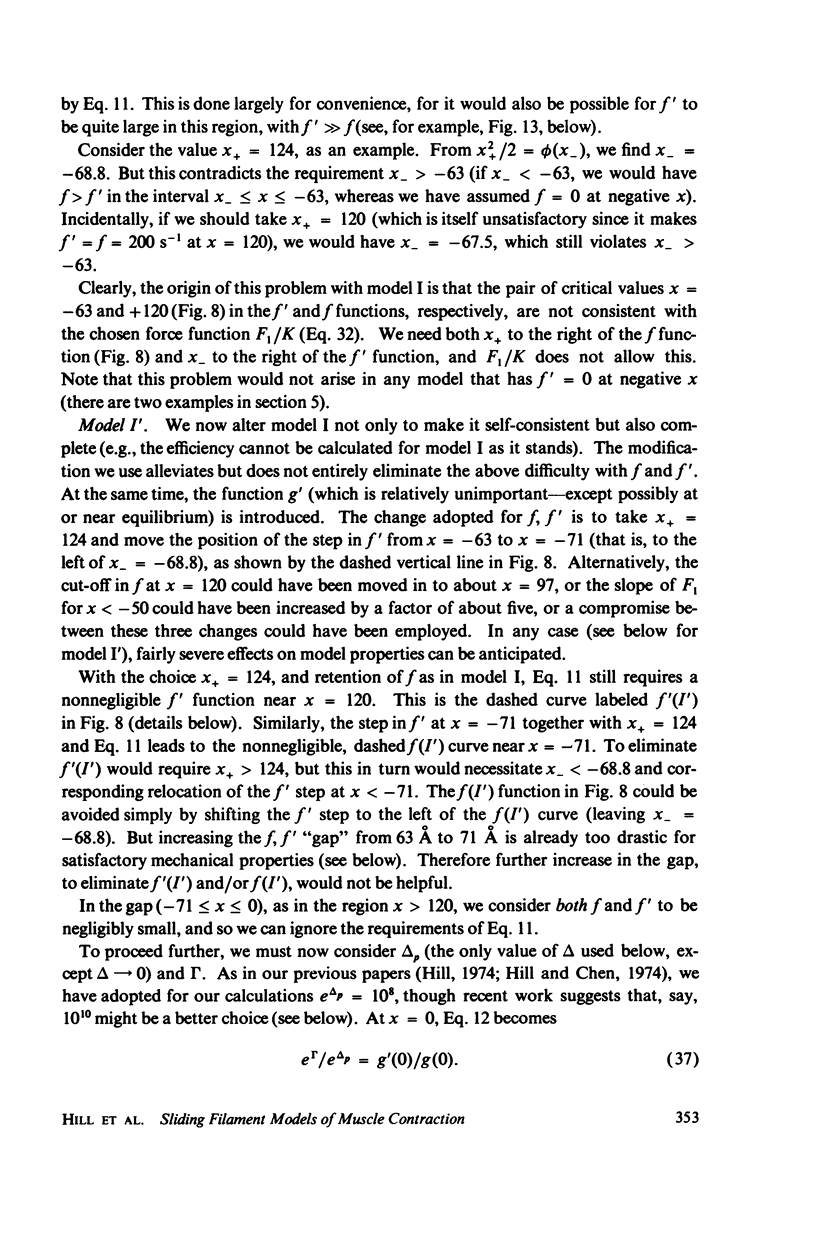
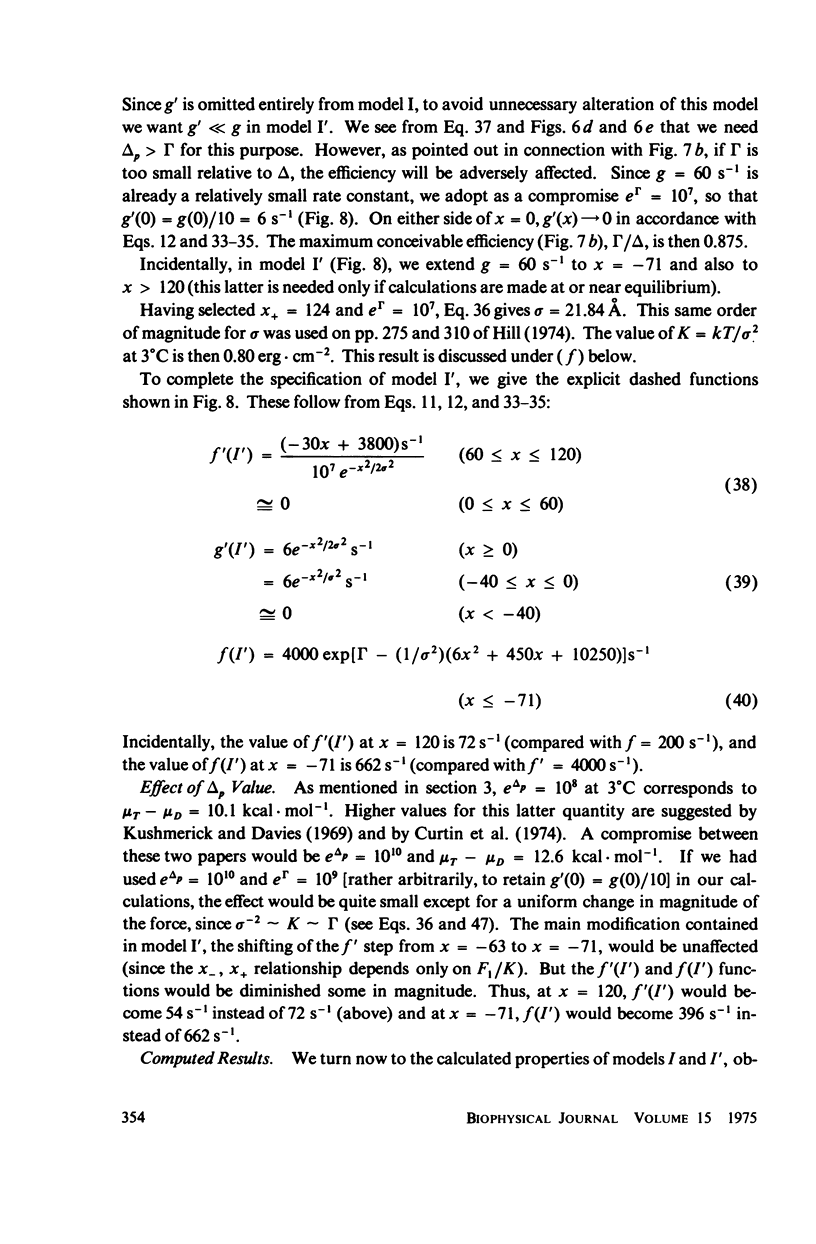
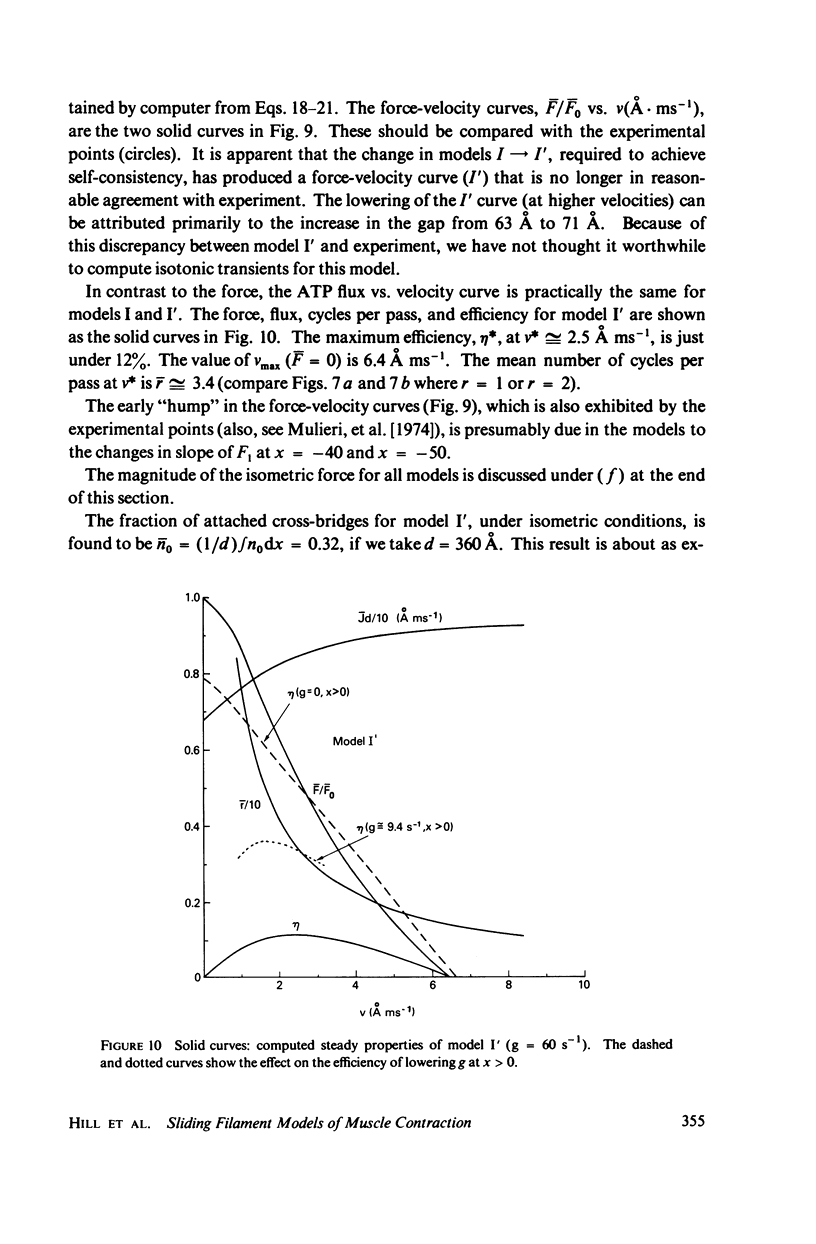
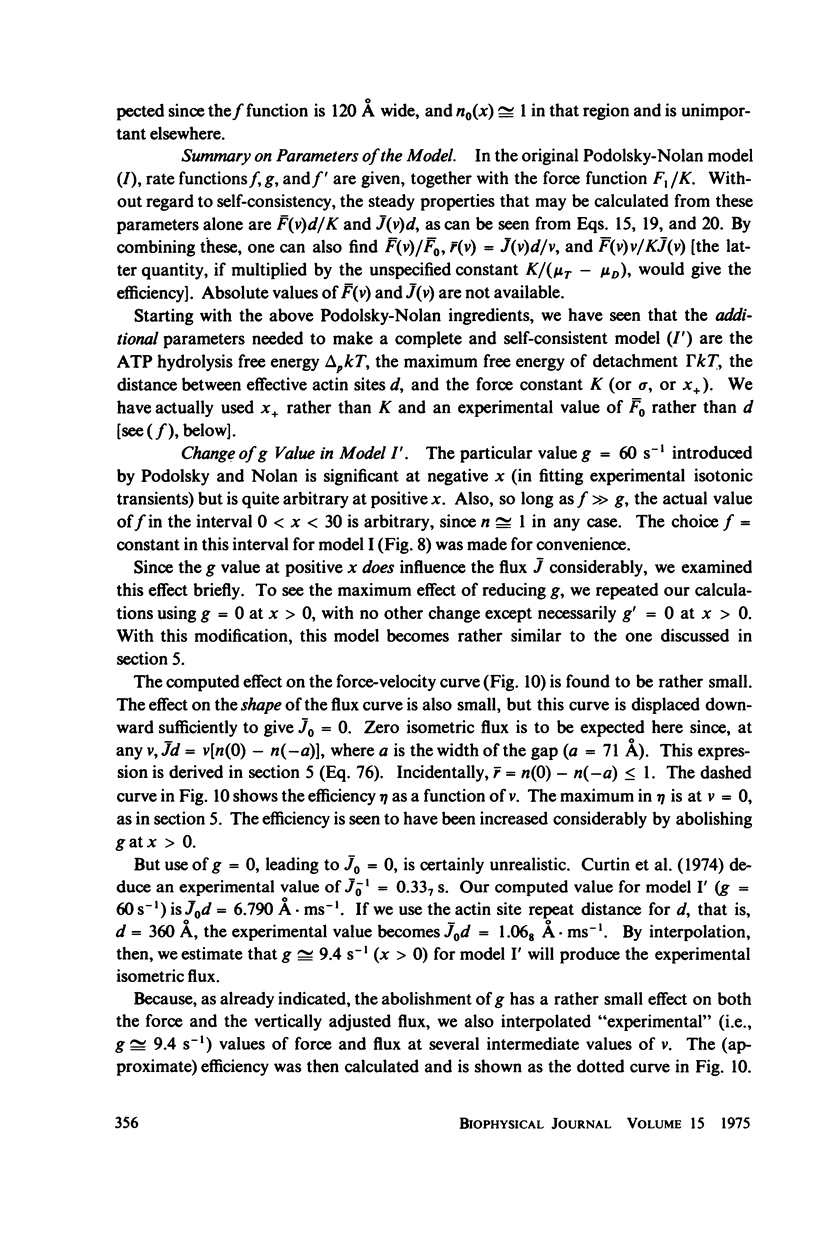
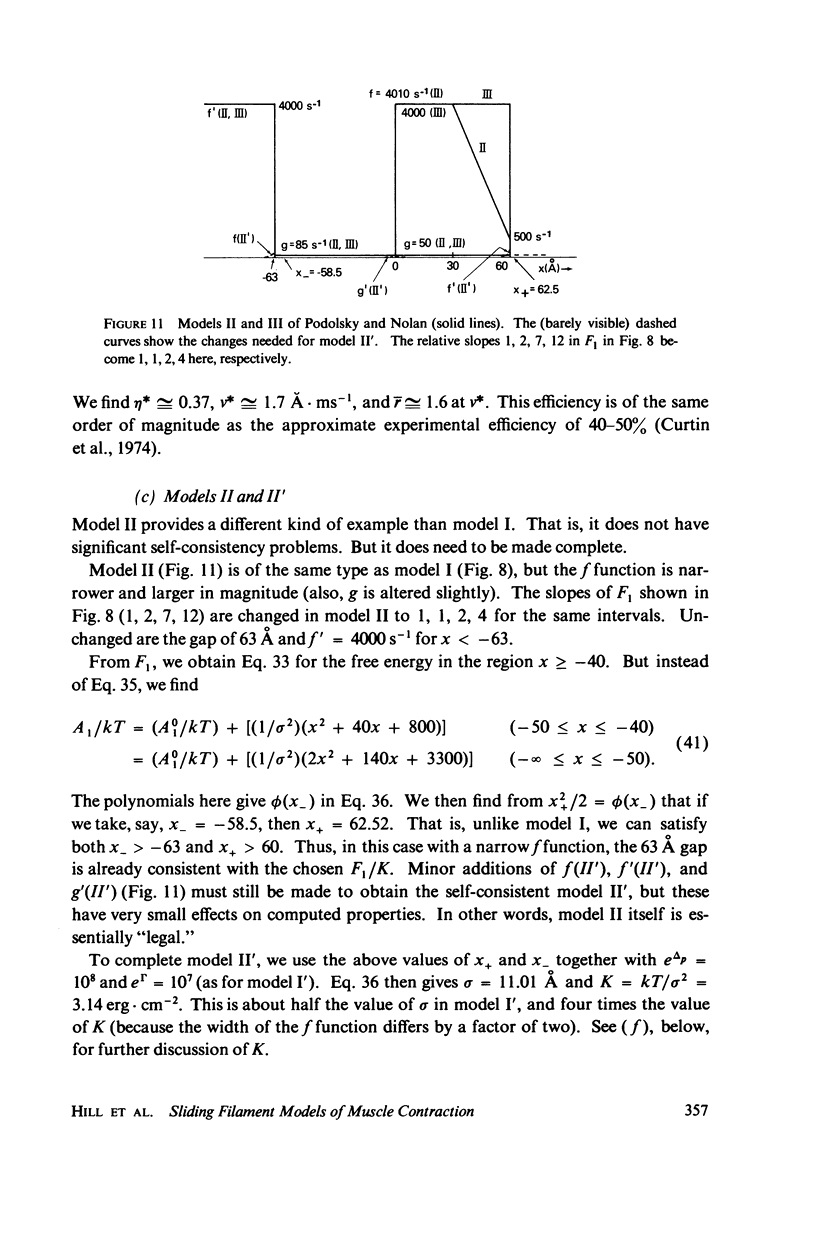
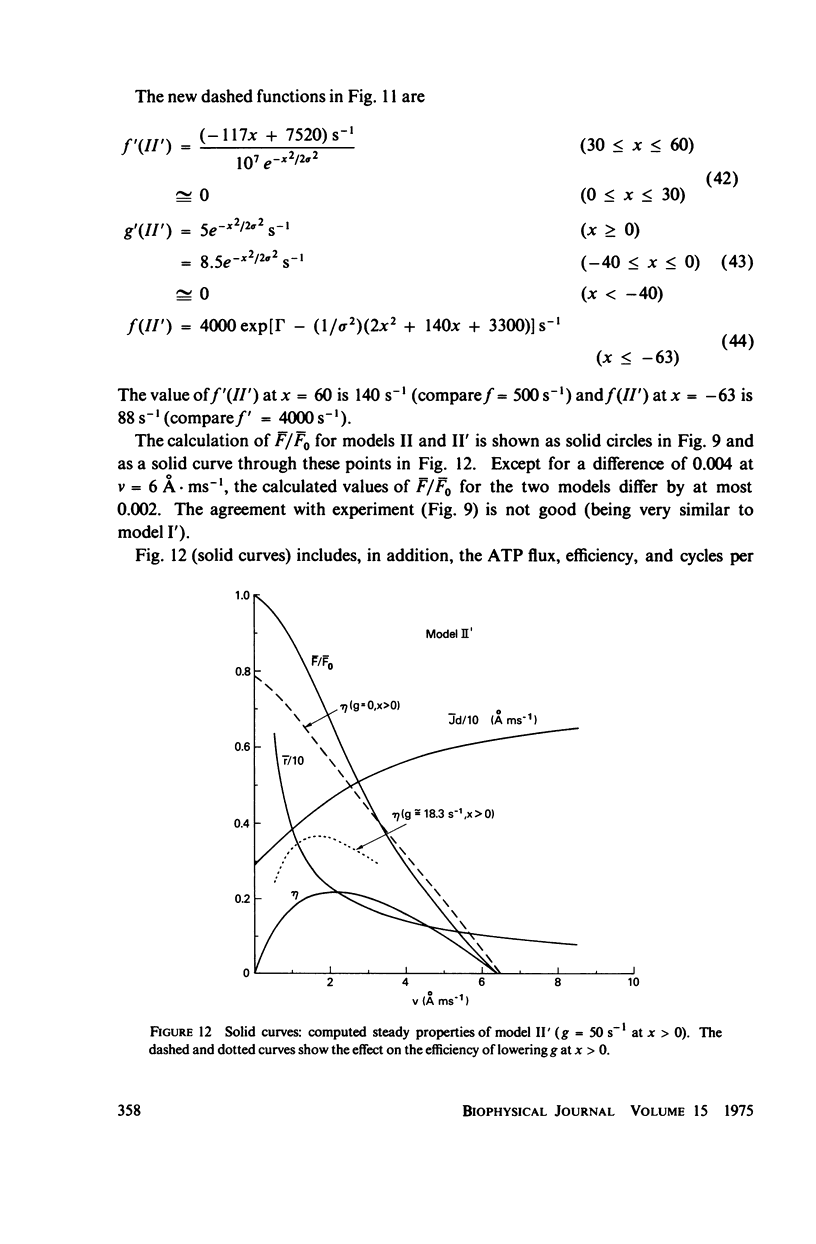
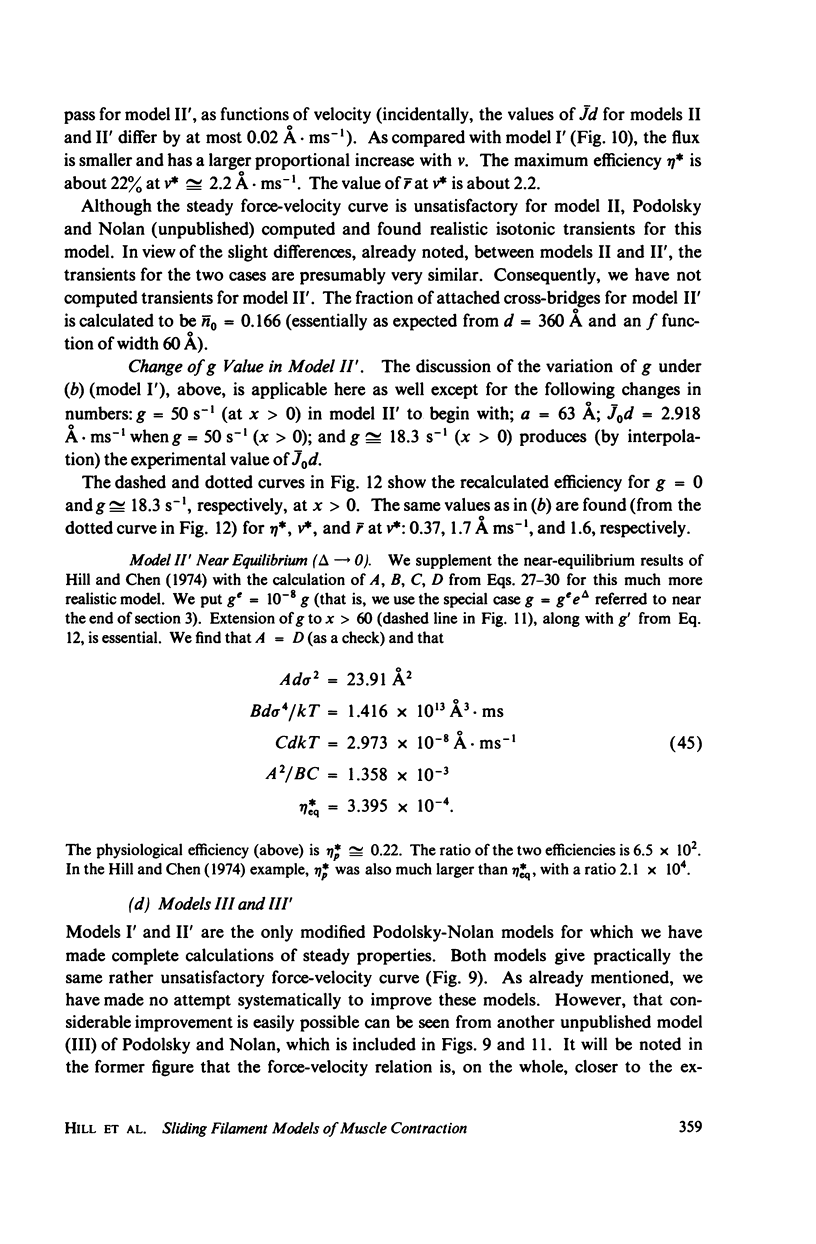
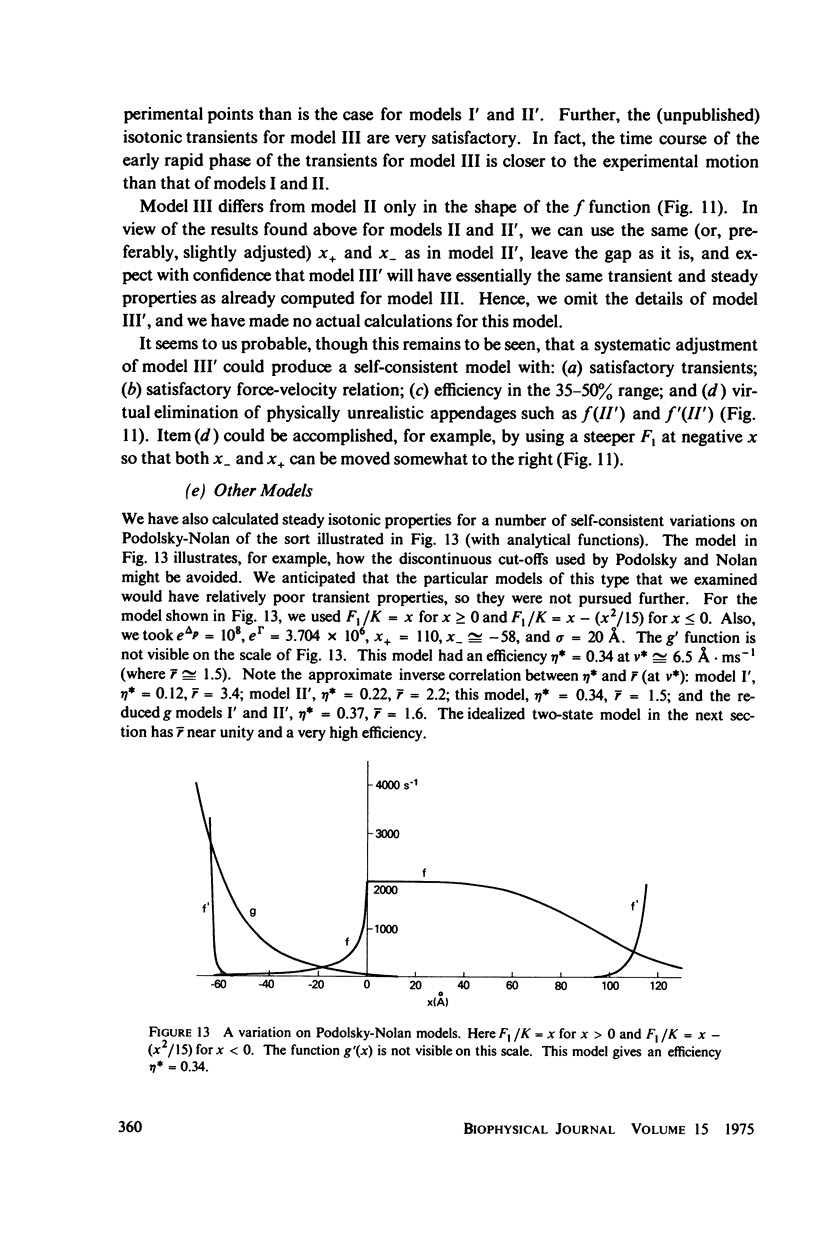
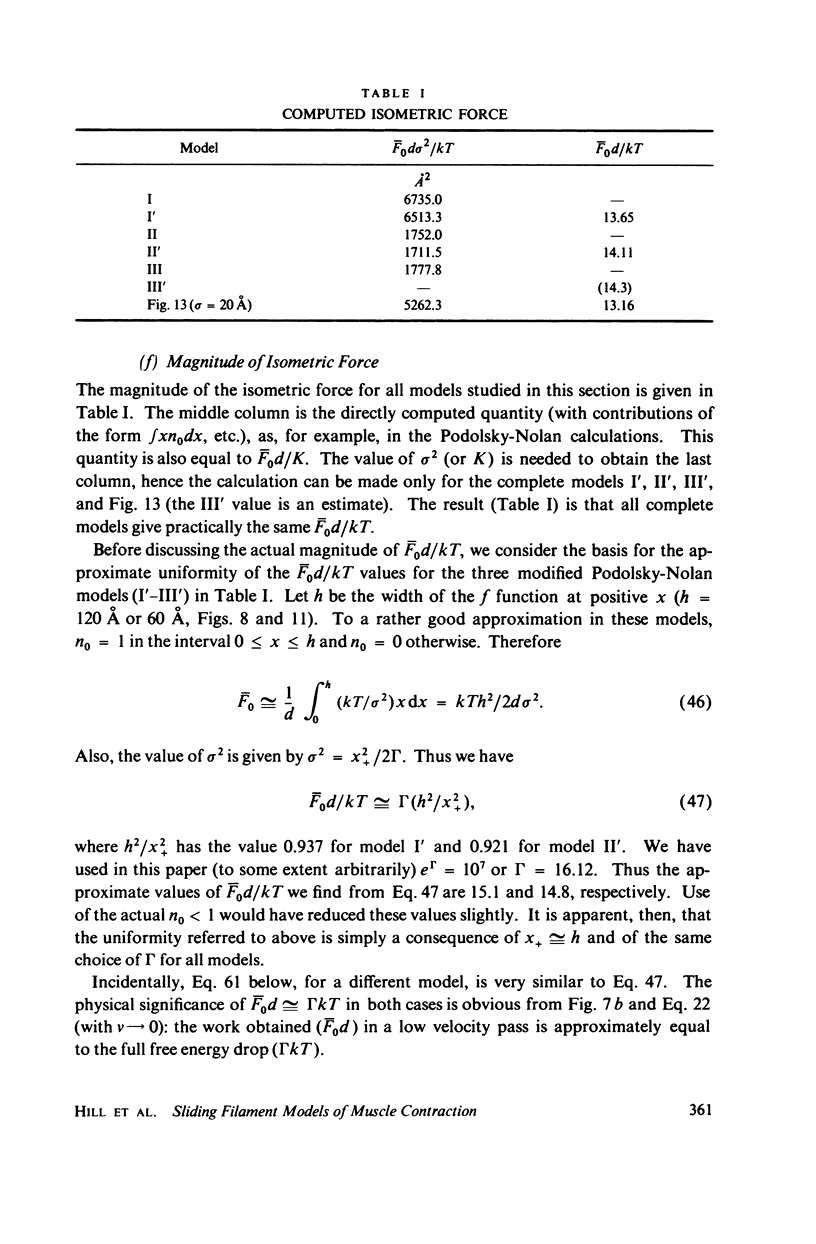
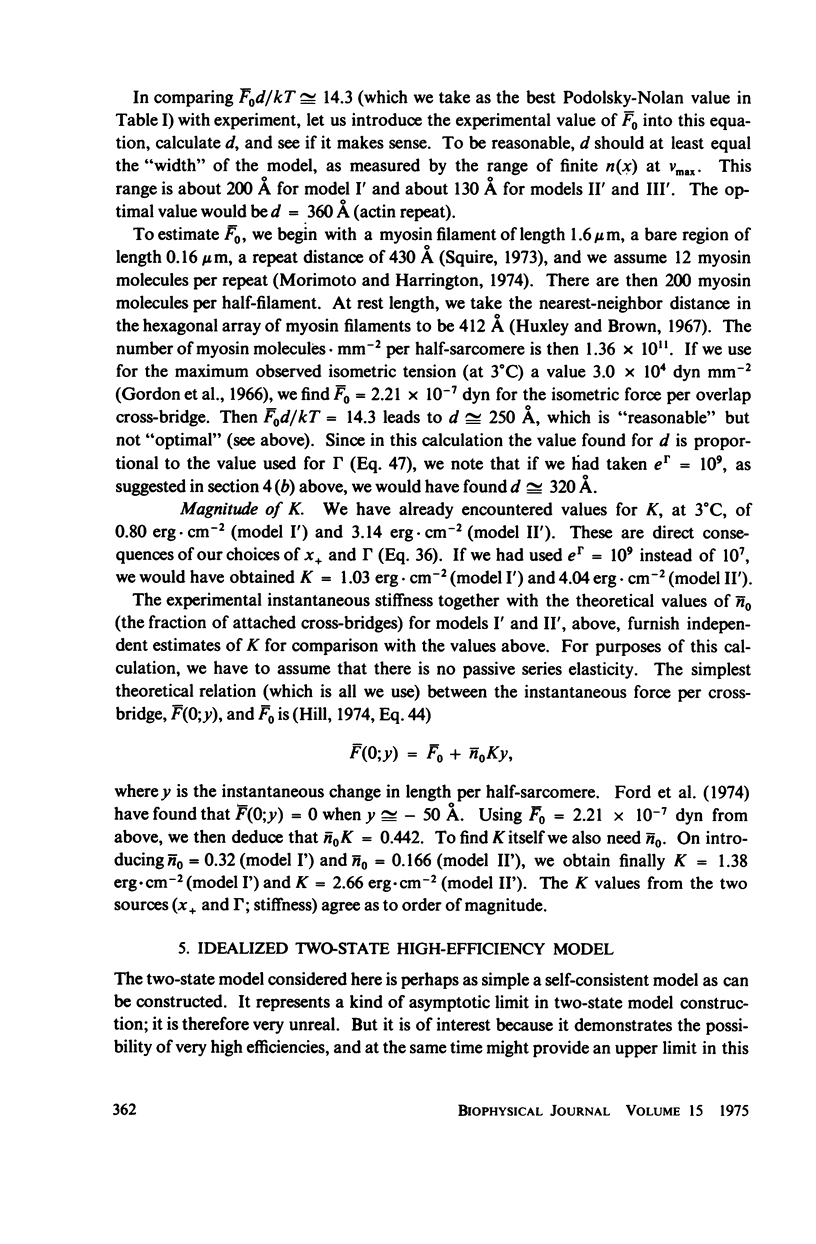
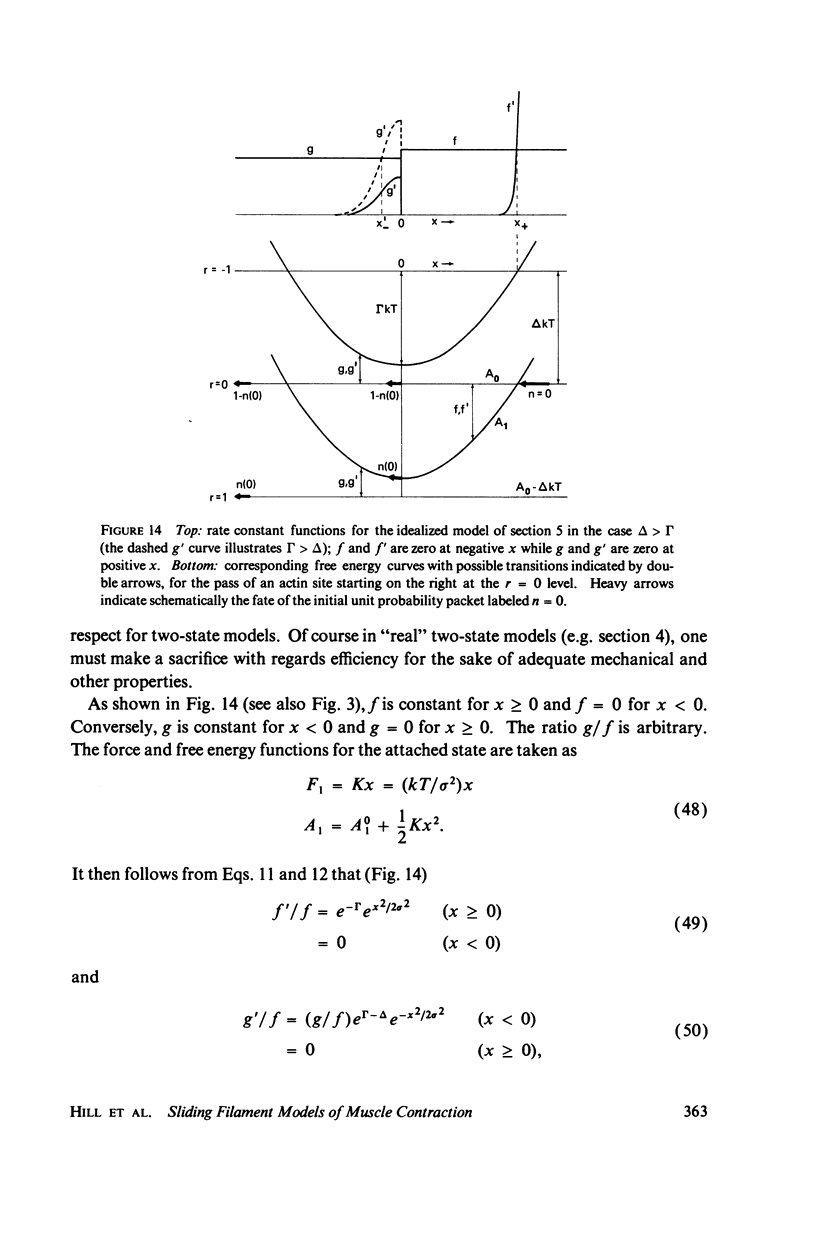
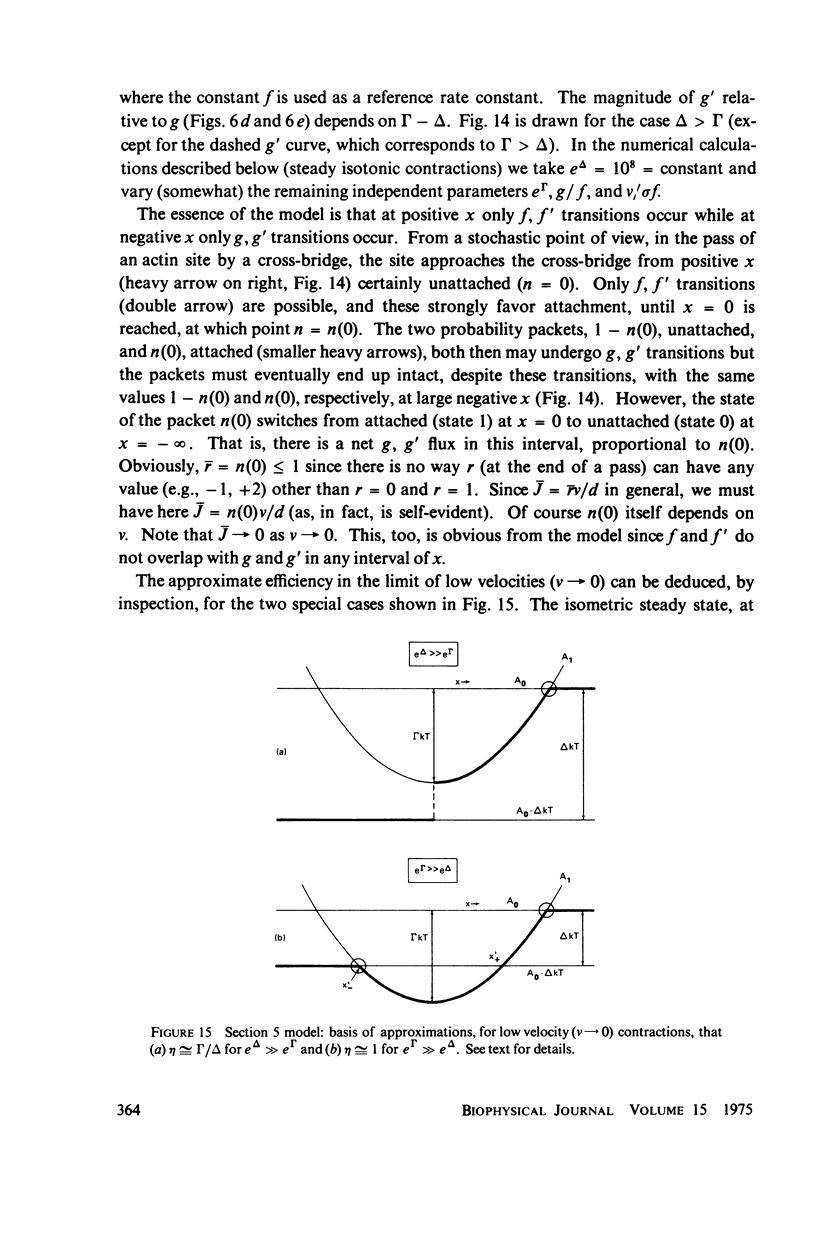
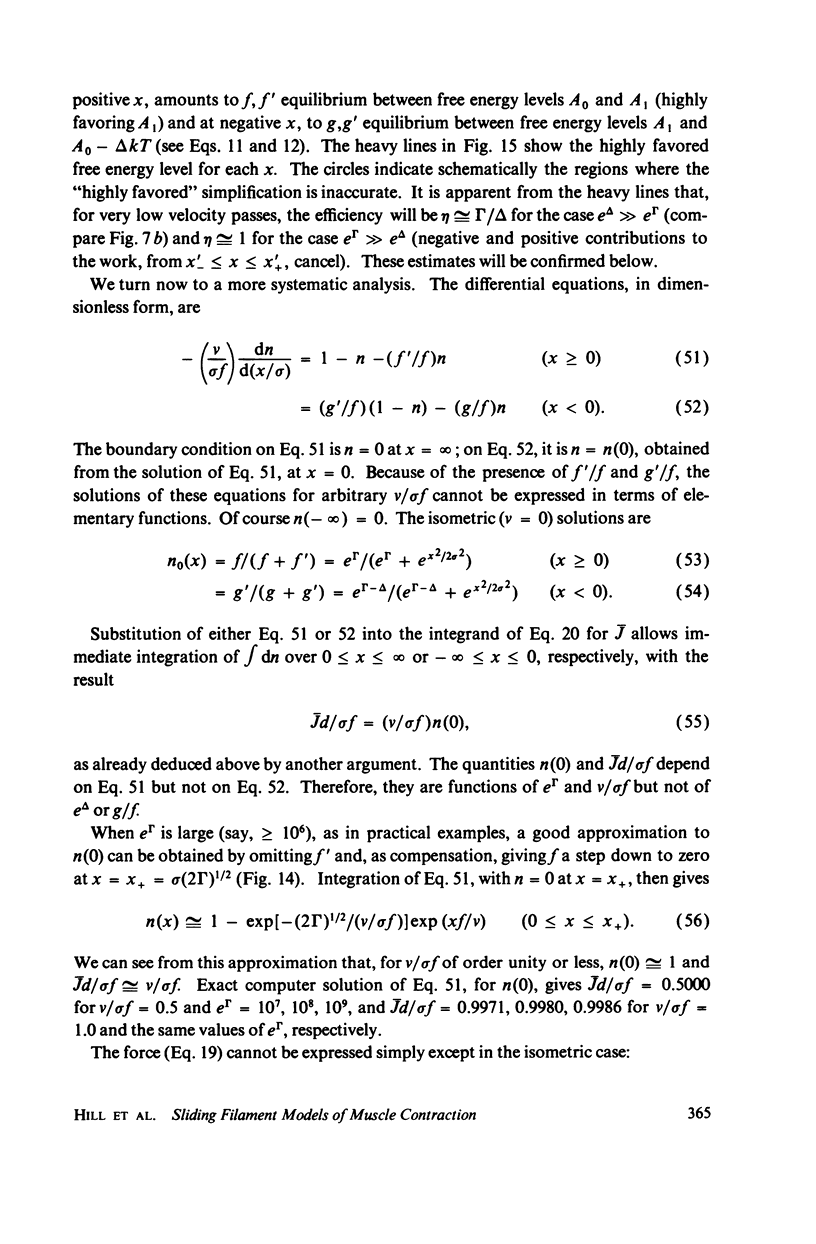
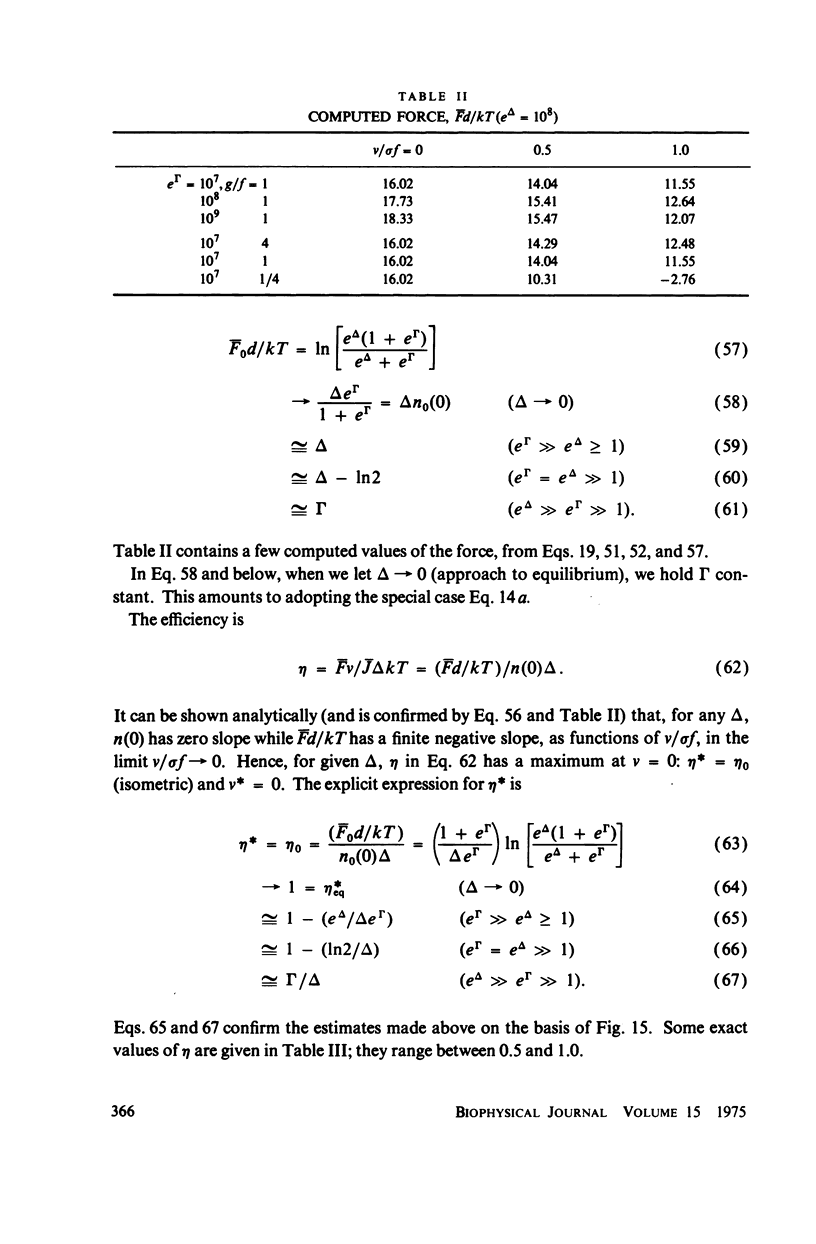
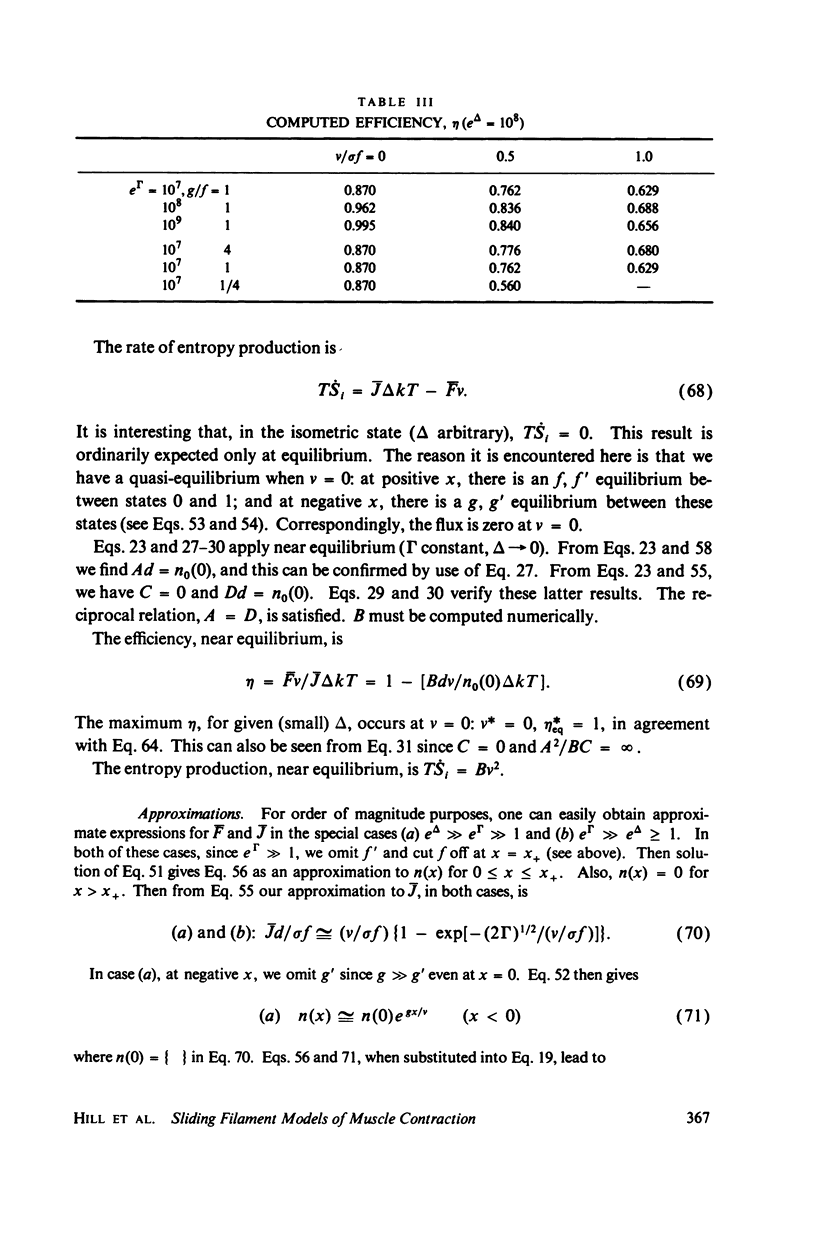
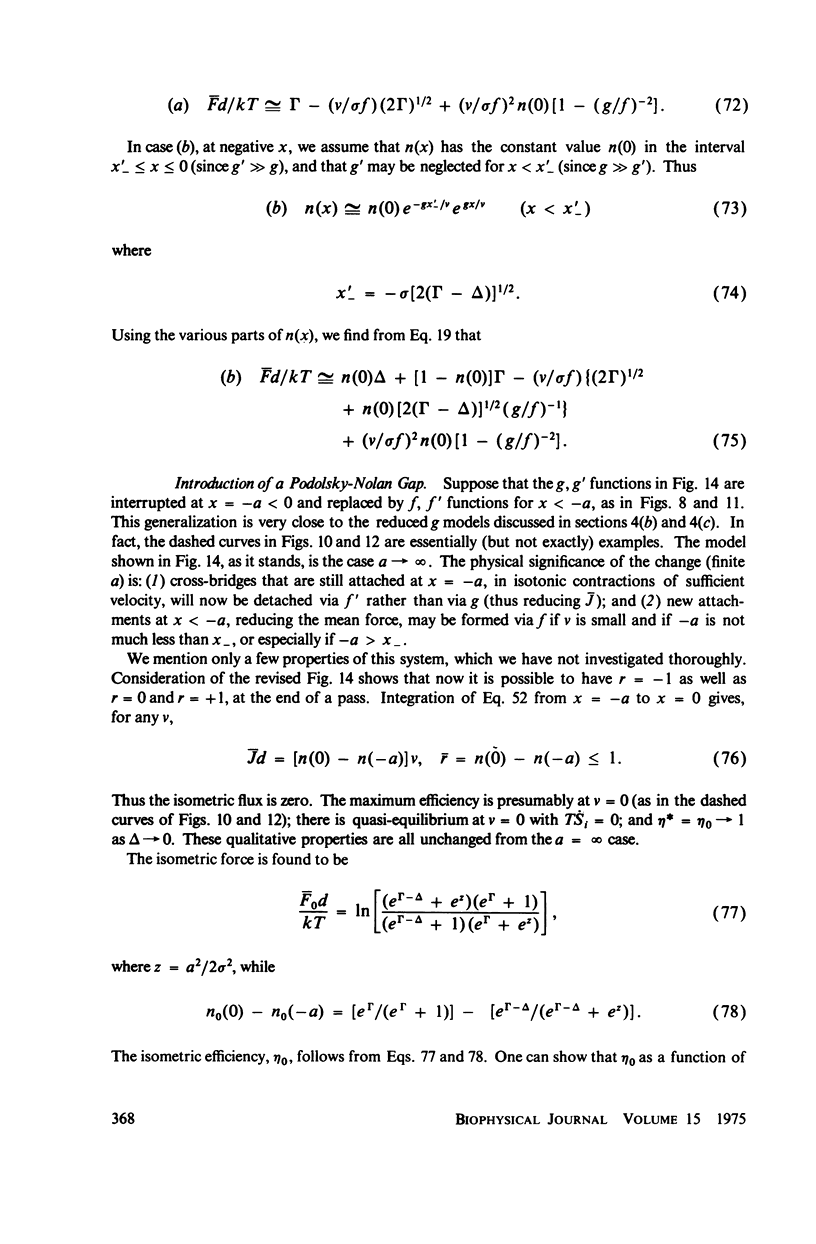
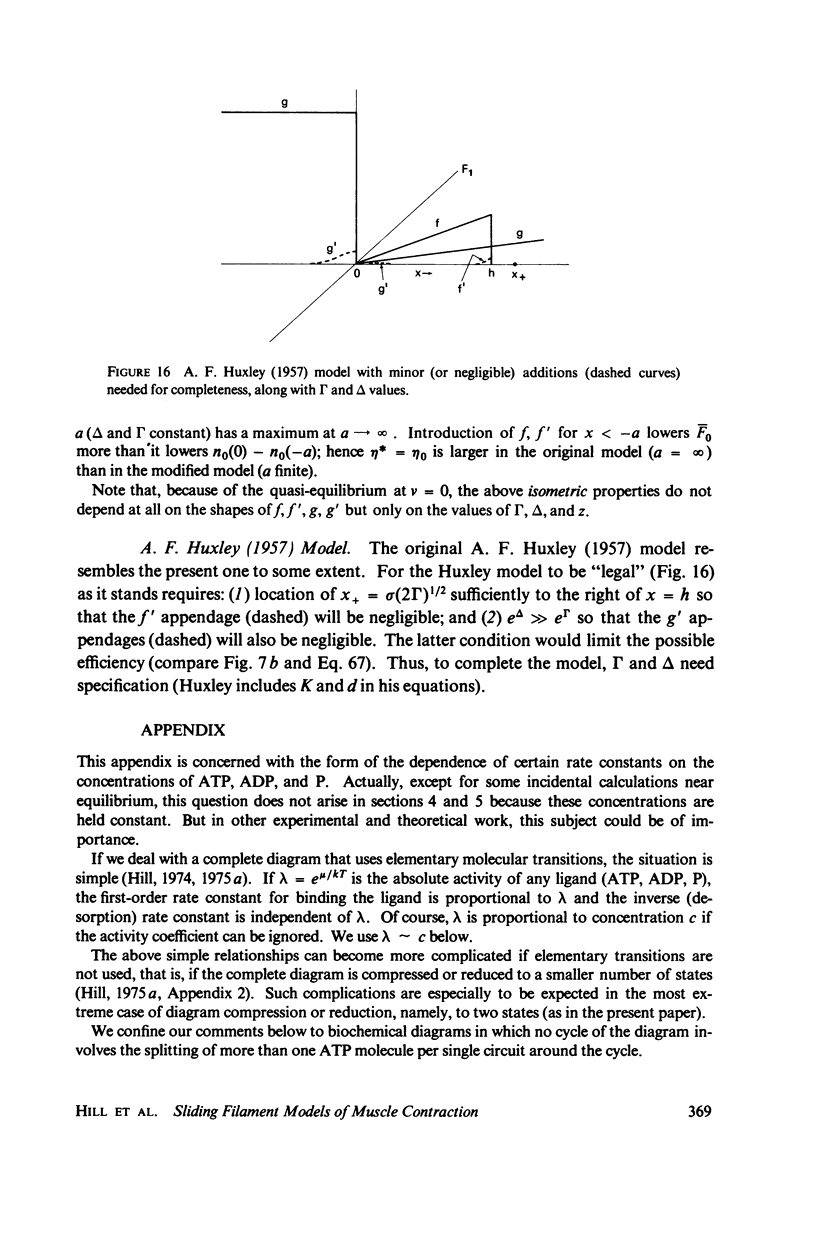
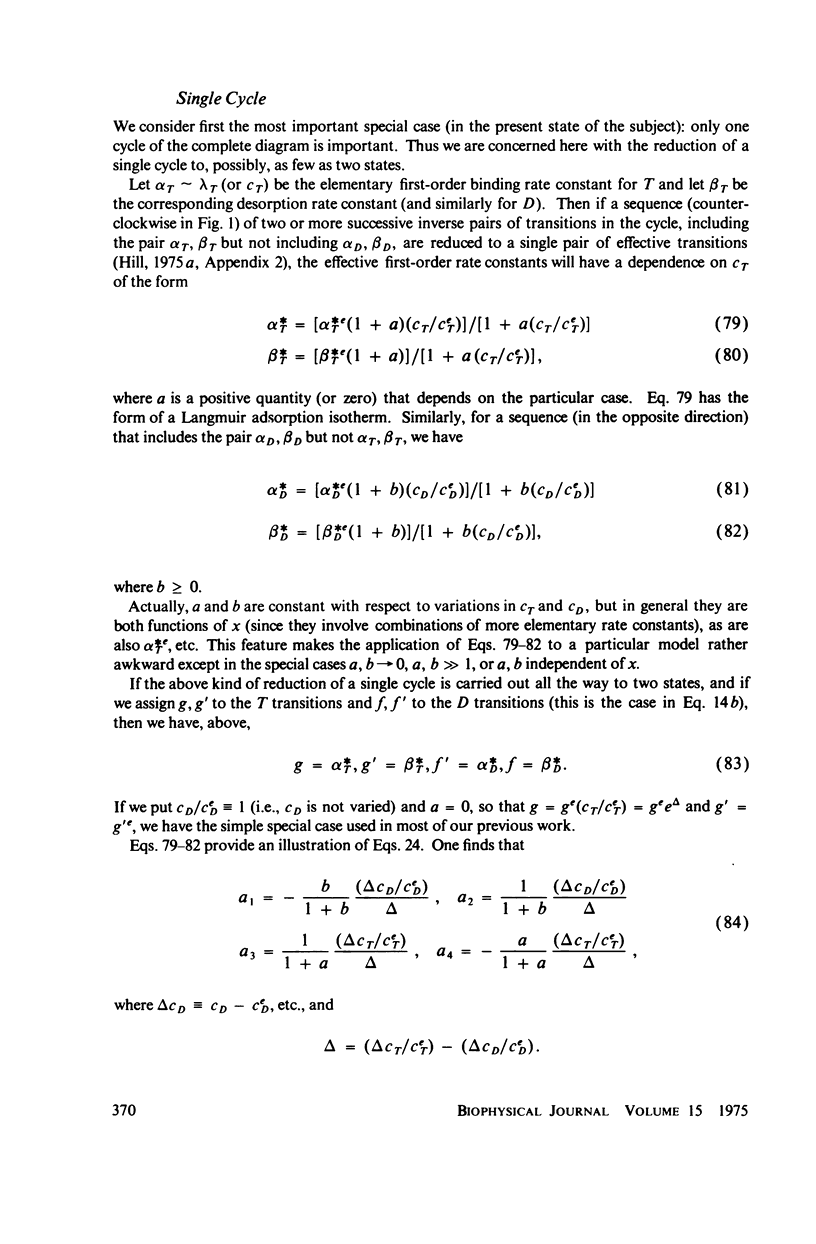
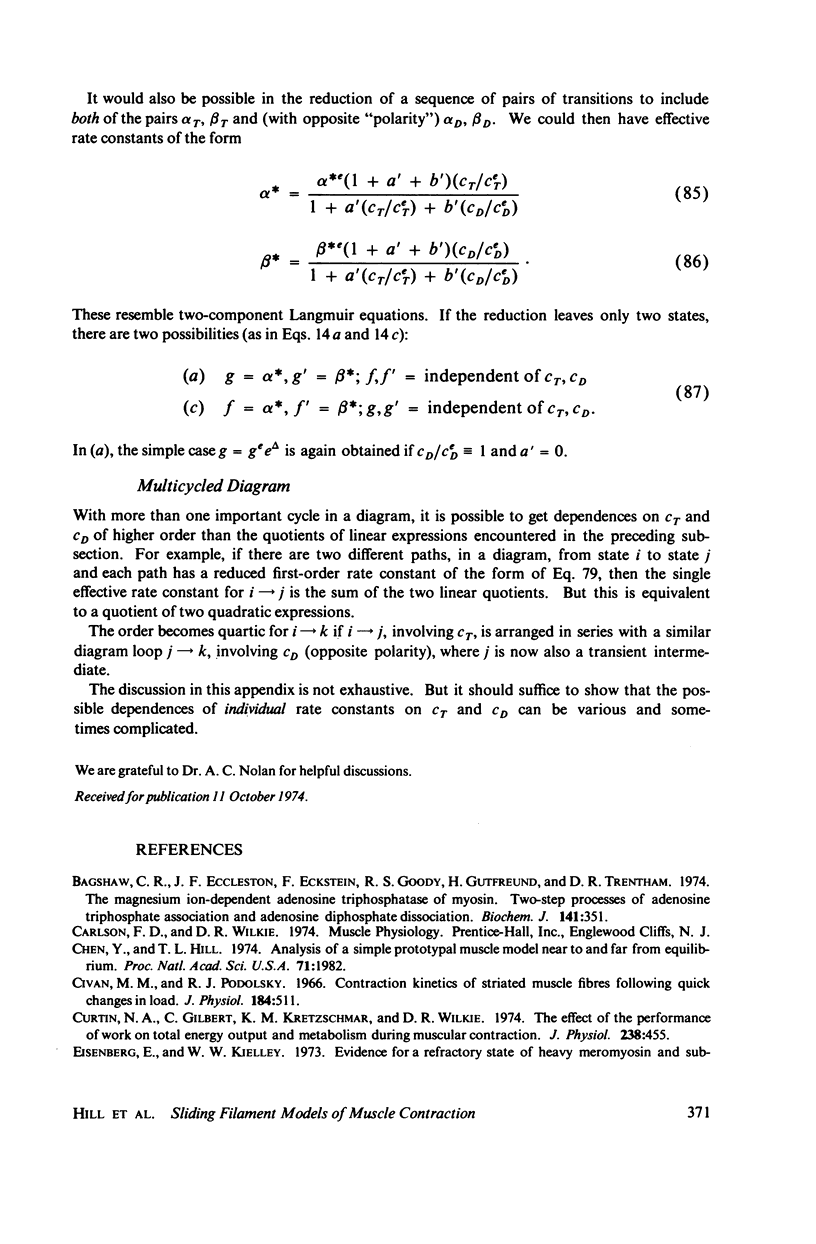
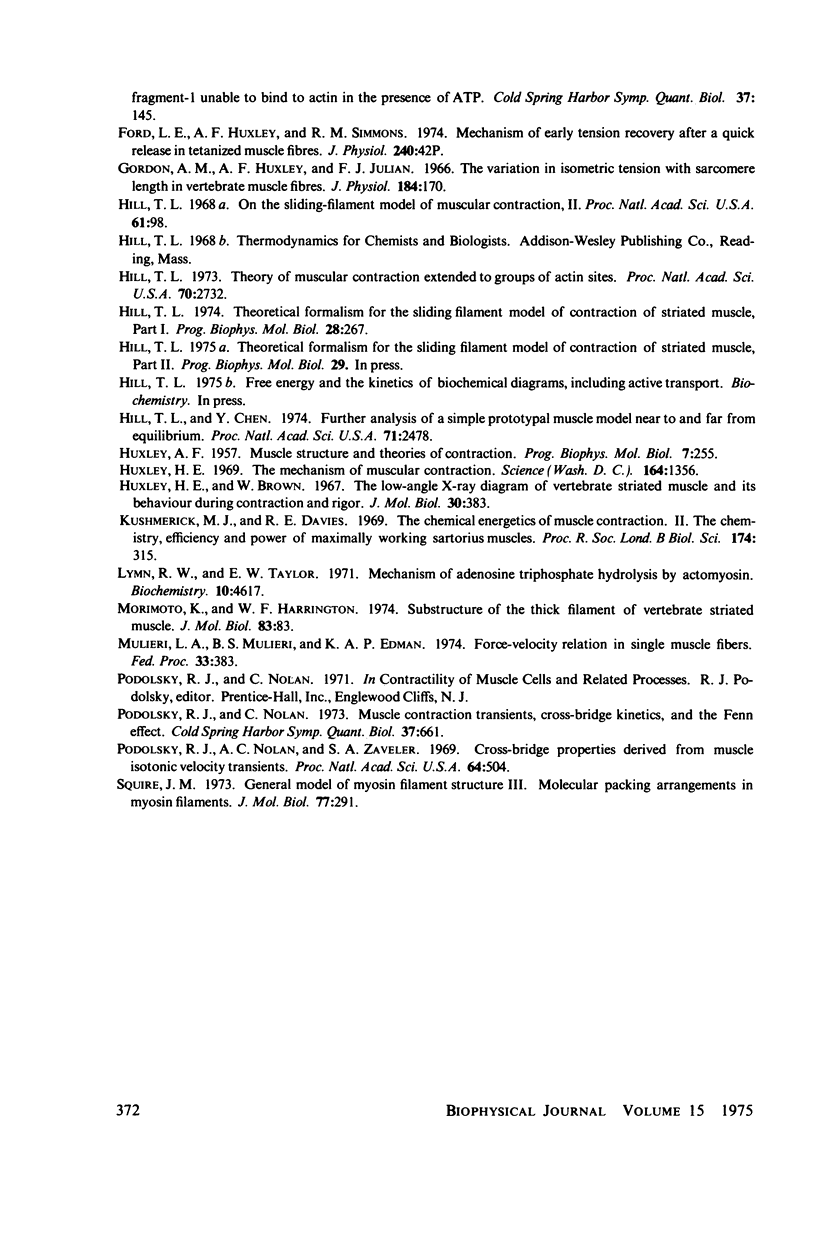
Selected References
These references are in PubMed. This may not be the complete list of references from this article.
- Bagshaw C. R., Eccleston J. F., Eckstein F., Goody R. S., Gutfreund H., Trentham D. R. The magnesium ion-dependent adenosine triphosphatase of myosin. Two-step processes of adenosine triphosphate association and adenosine diphosphate dissociation. Biochem J. 1974 Aug;141(2):351–364. doi: 10.1042/bj1410351. [DOI] [PMC free article] [PubMed] [Google Scholar]
- Chen Y. D., Hill T. L. Analysis of a simple prototypal muscle model near to and far from equilibrium. Proc Natl Acad Sci U S A. 1974 May;71(5):1982–1986. doi: 10.1073/pnas.71.5.1982. [DOI] [PMC free article] [PubMed] [Google Scholar]
- Curtin N. A., Gilbert C., Kretzschmar K. M., Wilkie D. R. The effect of the performance of work on total energy output and metabolism during muscular contraction. J Physiol. 1974 May;238(3):455–472. doi: 10.1113/jphysiol.1974.sp010537. [DOI] [PMC free article] [PubMed] [Google Scholar]
- Ford L. E., Huxley A. F., Simmons R. M. Proceedings: Mechanism of early tension recovery after a quick release in tetanized muscle fibres. J Physiol. 1974 Jul;240(2):42P–43P. [PubMed] [Google Scholar]
- Gordon A. M., Huxley A. F., Julian F. J. The variation in isometric tension with sarcomere length in vertebrate muscle fibres. J Physiol. 1966 May;184(1):170–192. doi: 10.1113/jphysiol.1966.sp007909. [DOI] [PMC free article] [PubMed] [Google Scholar]
- HUXLEY A. F. Muscle structure and theories of contraction. Prog Biophys Biophys Chem. 1957;7:255–318. [PubMed] [Google Scholar]
- Hill T. L., Chen Y. D. Further analysis of a simple prototypal muscle model near to and far from equilibrium. Proc Natl Acad Sci U S A. 1974 Jun;71(6):2478–2481. doi: 10.1073/pnas.71.6.2478. [DOI] [PMC free article] [PubMed] [Google Scholar]
- Hill T. L. Theoretical formalism for the sliding filament model of contraction of striated muscle. Part I. Prog Biophys Mol Biol. 1974;28:267–340. doi: 10.1016/0079-6107(74)90020-0. [DOI] [PubMed] [Google Scholar]
- Hill T. L. Theory of muscular contraction extended to groups of actin sites. Proc Natl Acad Sci U S A. 1973 Oct;70(10):2732–2736. doi: 10.1073/pnas.70.10.2732. [DOI] [PMC free article] [PubMed] [Google Scholar]
- Huxley H. E., Brown W. The low-angle x-ray diagram of vertebrate striated muscle and its behaviour during contraction and rigor. J Mol Biol. 1967 Dec 14;30(2):383–434. doi: 10.1016/s0022-2836(67)80046-9. [DOI] [PubMed] [Google Scholar]
- Huxley H. E. The mechanism of muscular contraction. Science. 1969 Jun 20;164(3886):1356–1365. doi: 10.1126/science.164.3886.1356. [DOI] [PubMed] [Google Scholar]
- Kushmerick M. J., Davies R. E. The chemical energetics of muscle contraction. II. The chemistry, efficiency and power of maximally working sartorius muscles. Appendix. Free energy and enthalpy of atp hydrolysis in the sarcoplasm. Proc R Soc Lond B Biol Sci. 1969 Dec 23;174(1036):315–353. doi: 10.1098/rspb.1969.0096. [DOI] [PubMed] [Google Scholar]
- Lymn R. W., Taylor E. W. Mechanism of adenosine triphosphate hydrolysis by actomyosin. Biochemistry. 1971 Dec 7;10(25):4617–4624. doi: 10.1021/bi00801a004. [DOI] [PubMed] [Google Scholar]
- Morimoto K., Harrington W. F. Substructure of the thick filament of vertebrate striated muscle. J Mol Biol. 1974 Feb 15;83(1):83–97. doi: 10.1016/0022-2836(74)90425-2. [DOI] [PubMed] [Google Scholar]
- Podolsky R. J., Nolan A. C., Zaveler S. A. Cross-bridge properties derived from muscle isotonic velocity transients. Proc Natl Acad Sci U S A. 1969 Oct;64(2):504–511. doi: 10.1073/pnas.64.2.504. [DOI] [PMC free article] [PubMed] [Google Scholar]
- Squire J. M. General model of myosin filament structure. 3. Molecular packing arrangements in myosin filaments. J Mol Biol. 1973 Jun 25;77(2):291–323. doi: 10.1016/0022-2836(73)90337-9. [DOI] [PubMed] [Google Scholar]