Abstract
We consider a model for voltage-dependent gating of channels in which the gating charges are on the channel wall and move only a small distance. When this movement occurs across the closed gate, the charges move through the entire transmembrane potential, which is energetically equivalent to their moving across the entire membrane. The channel exists in two open states, O1 and O2, and two closed states, C1 and C2; each open and closed configuration is divided into two states because of the two possible positions of the gating charges. An unusual property of this model is that the electrical work in going from an open to a closed configuration (for example, in going from O1 to C2) is path dependent, and net work can result from going reversibly around a complete cycle. The model channel, like many biological channels, shows bursting activity. This flickering on and off of the channel enables the gate to sense the electric field and decide if it should be in the open or closed configuration. We prove here some general theorms concerning the electrical work associated with the movements of the walls of channels and the movements of charges on these walls.
Full text
PDF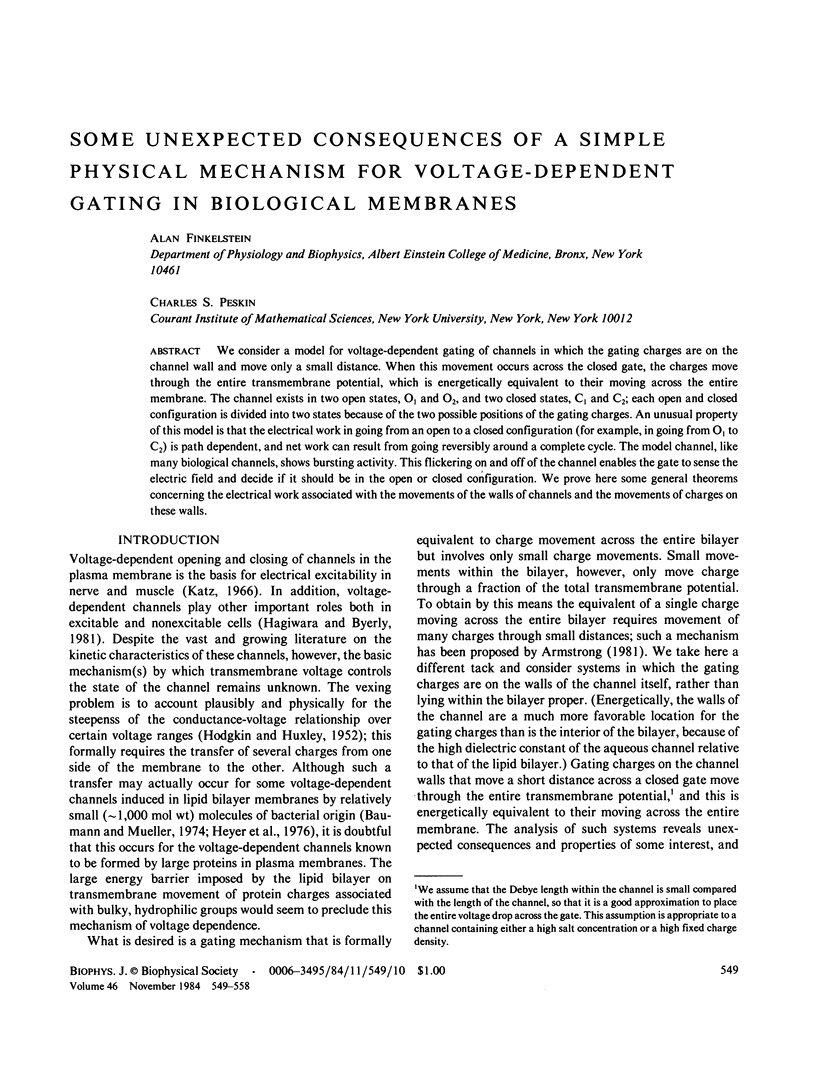
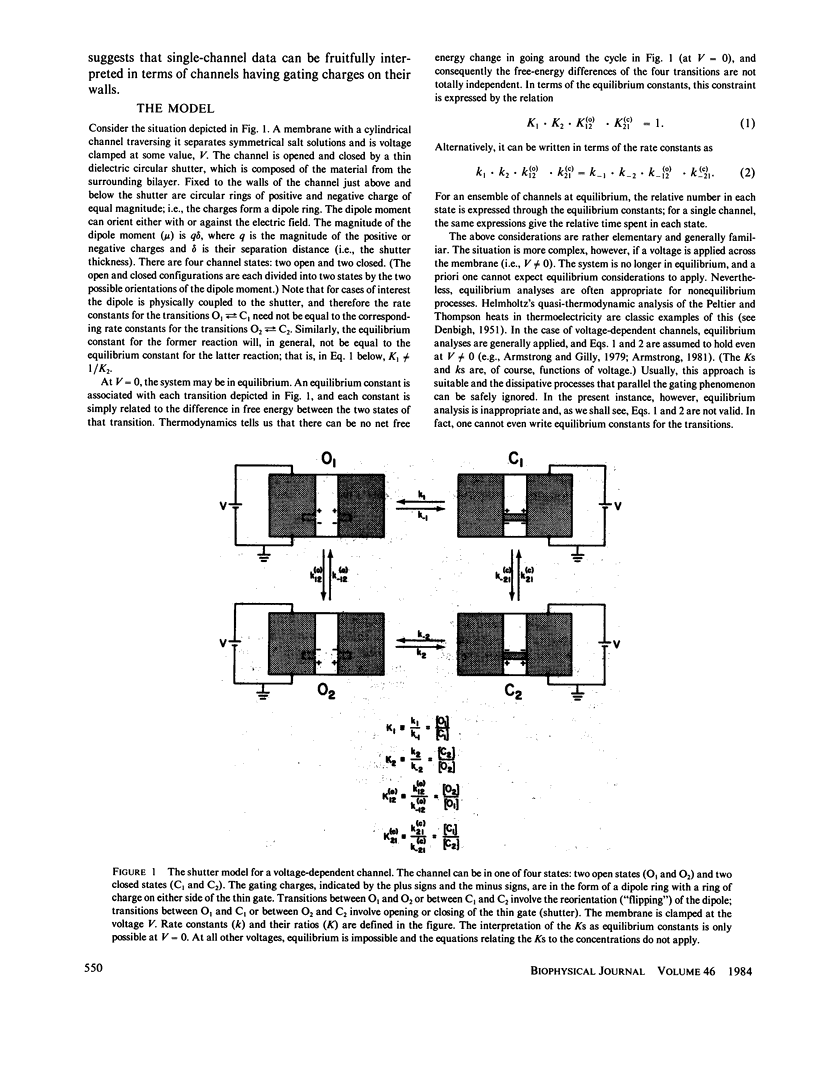
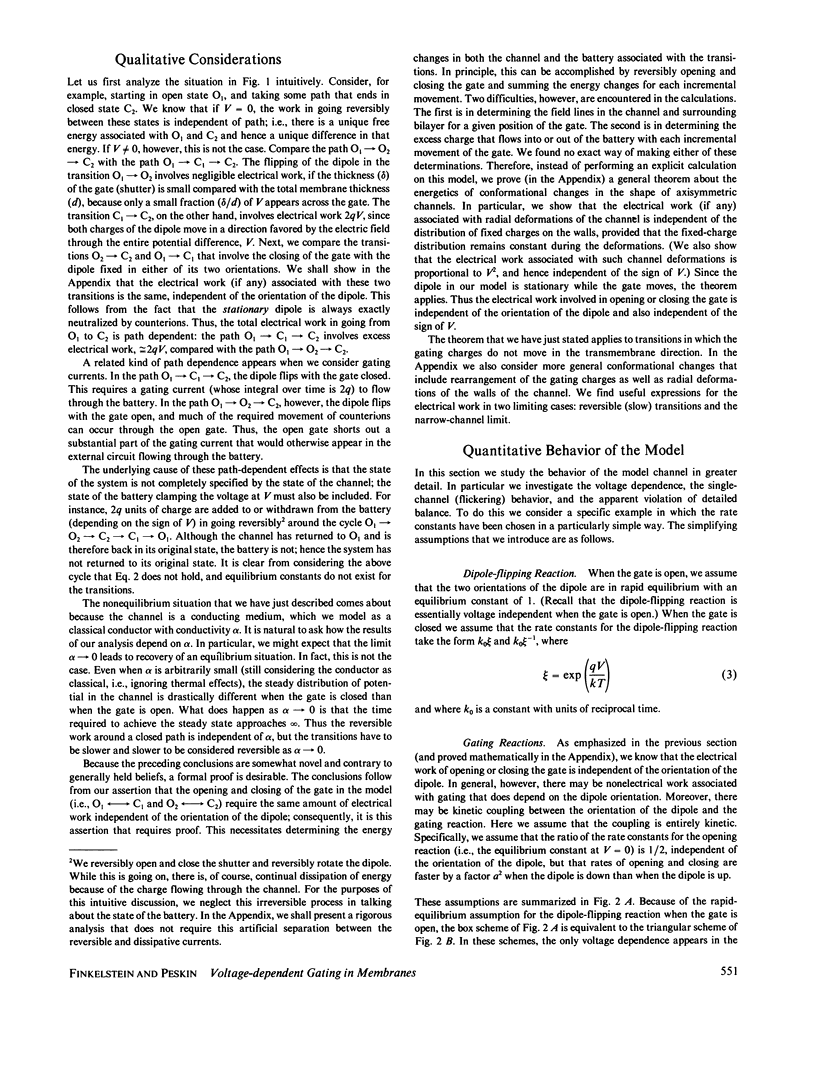
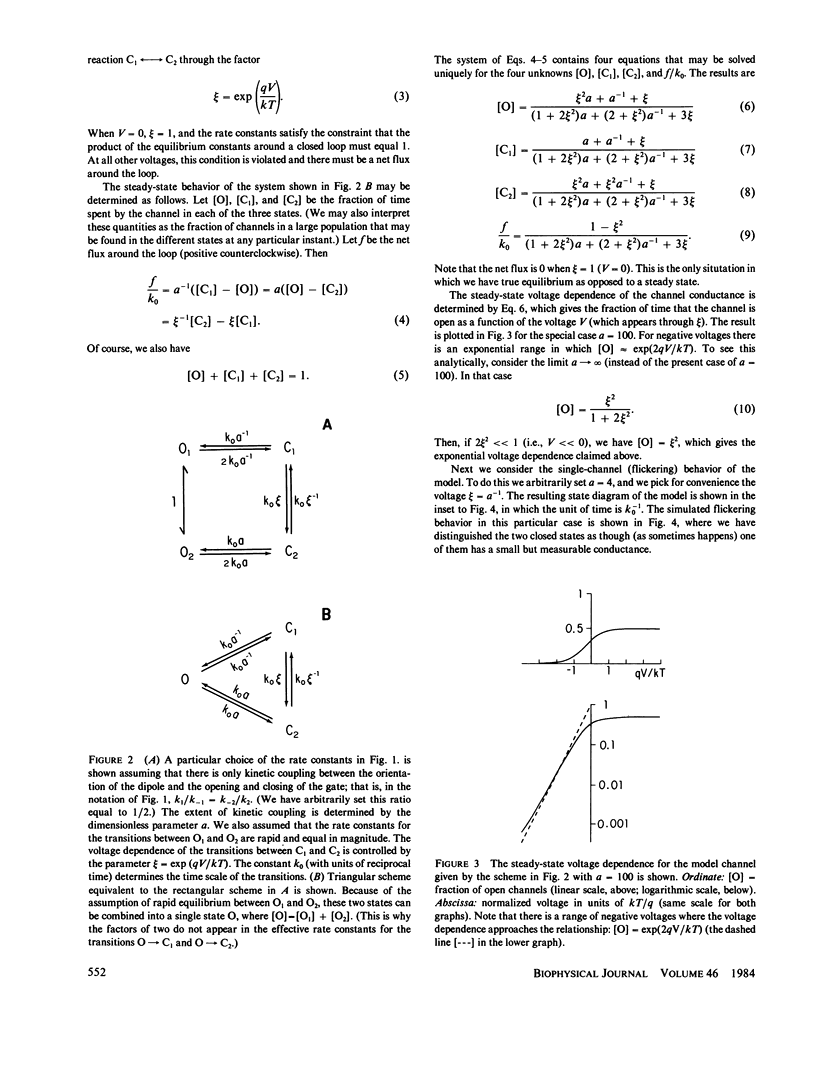
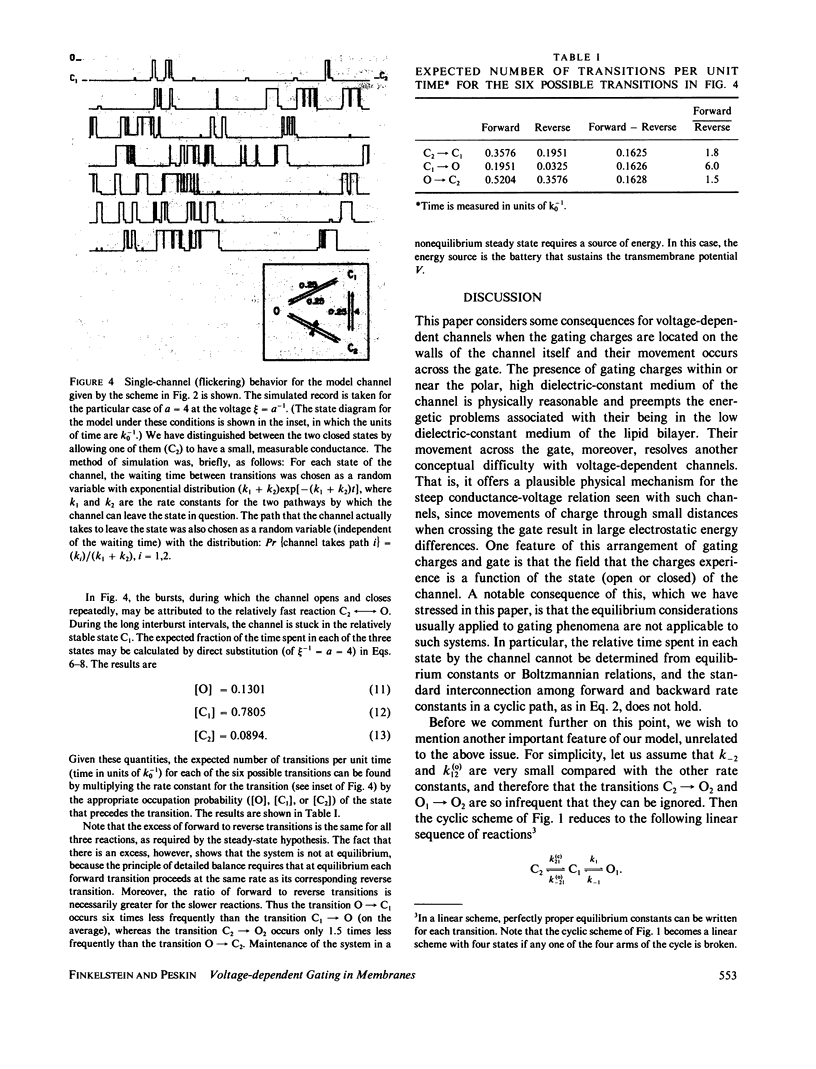
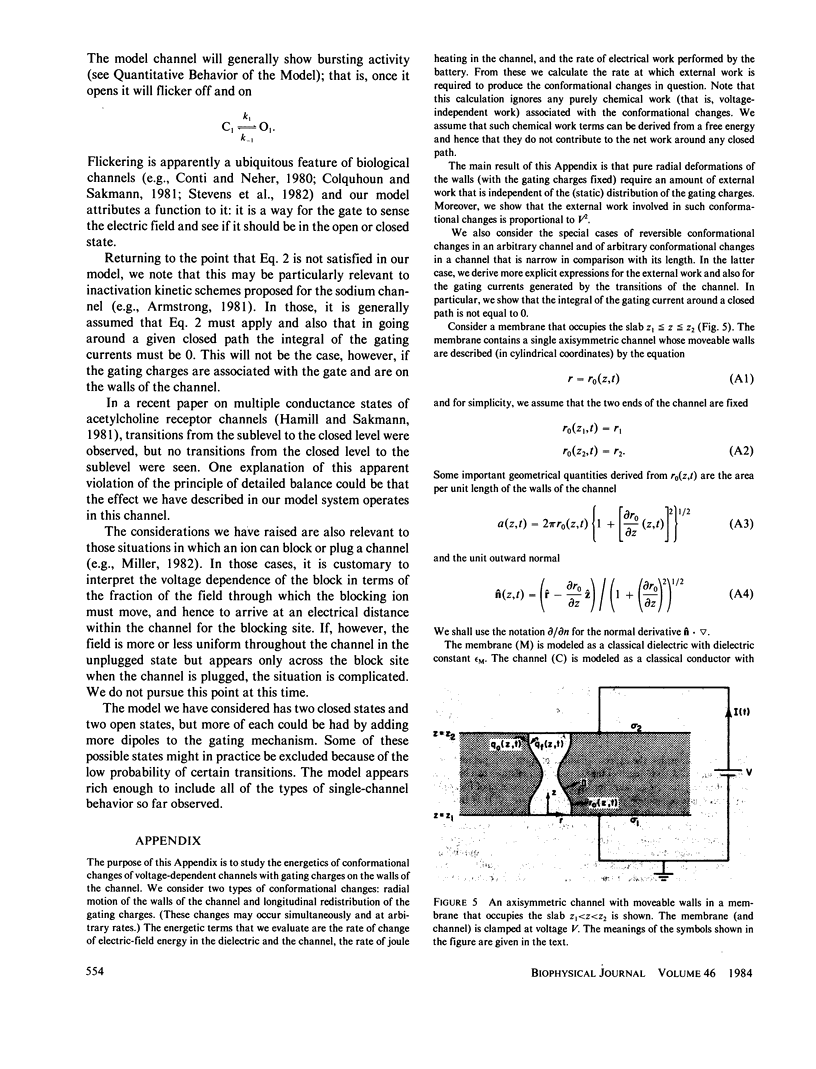
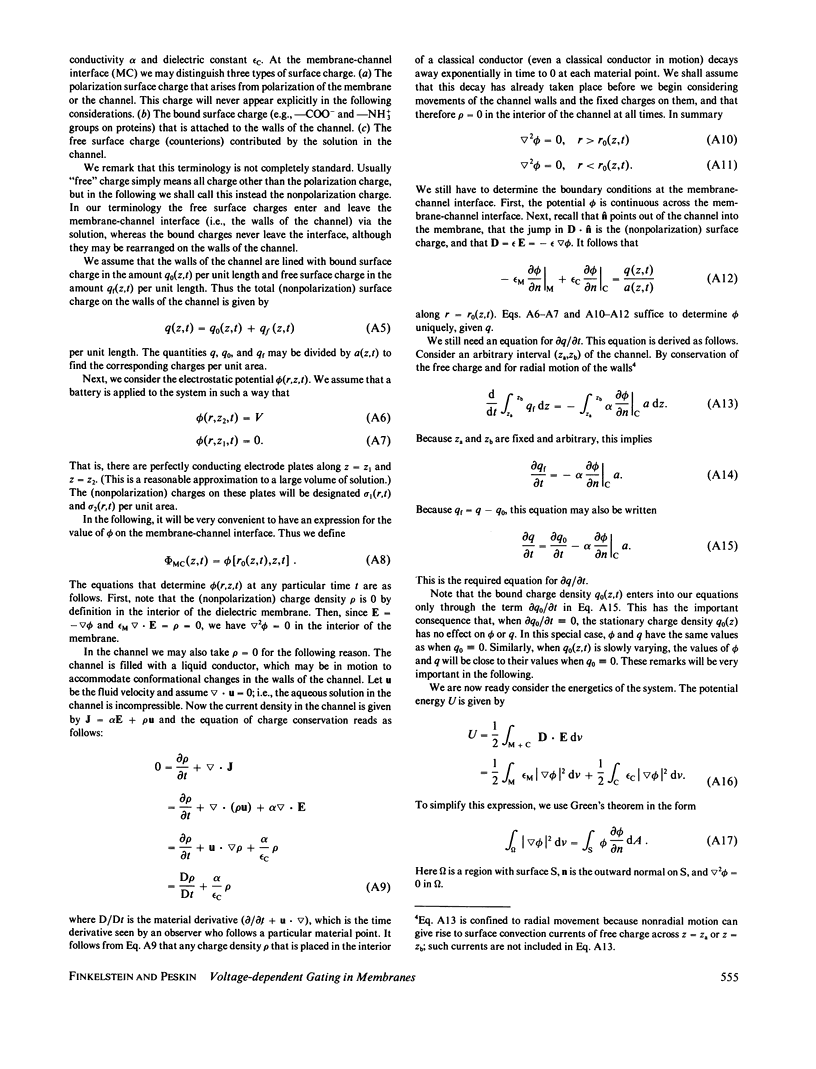
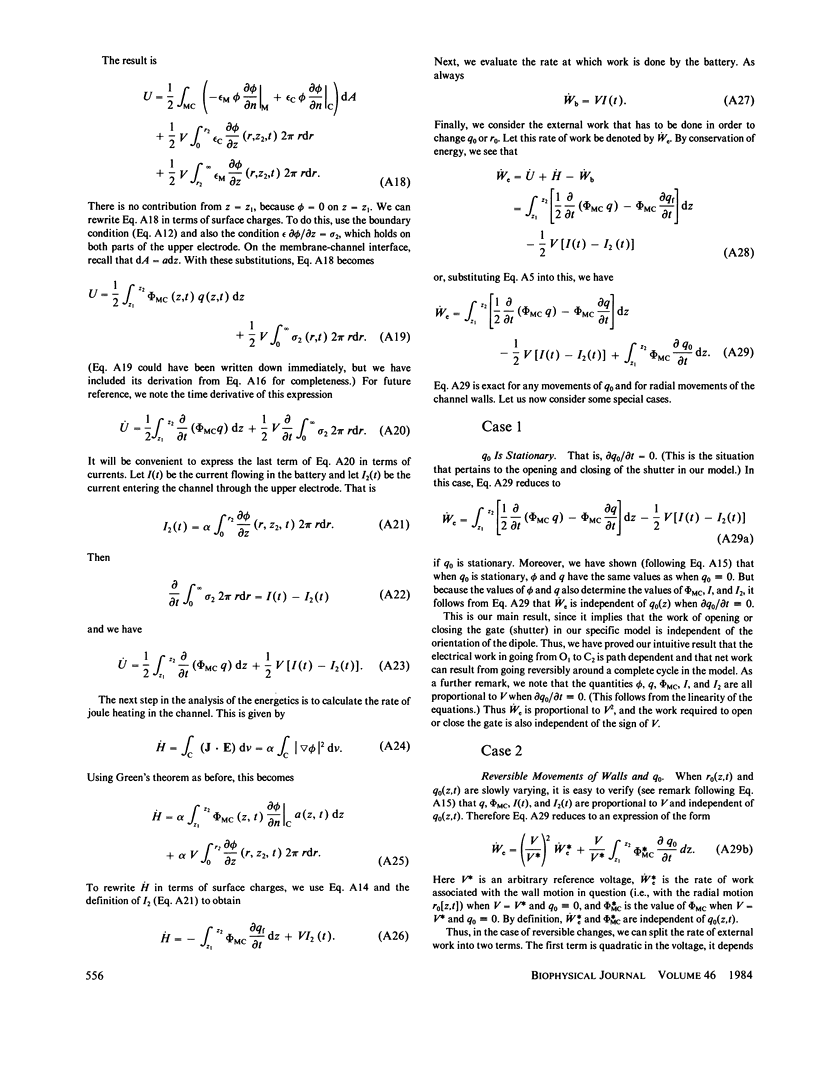
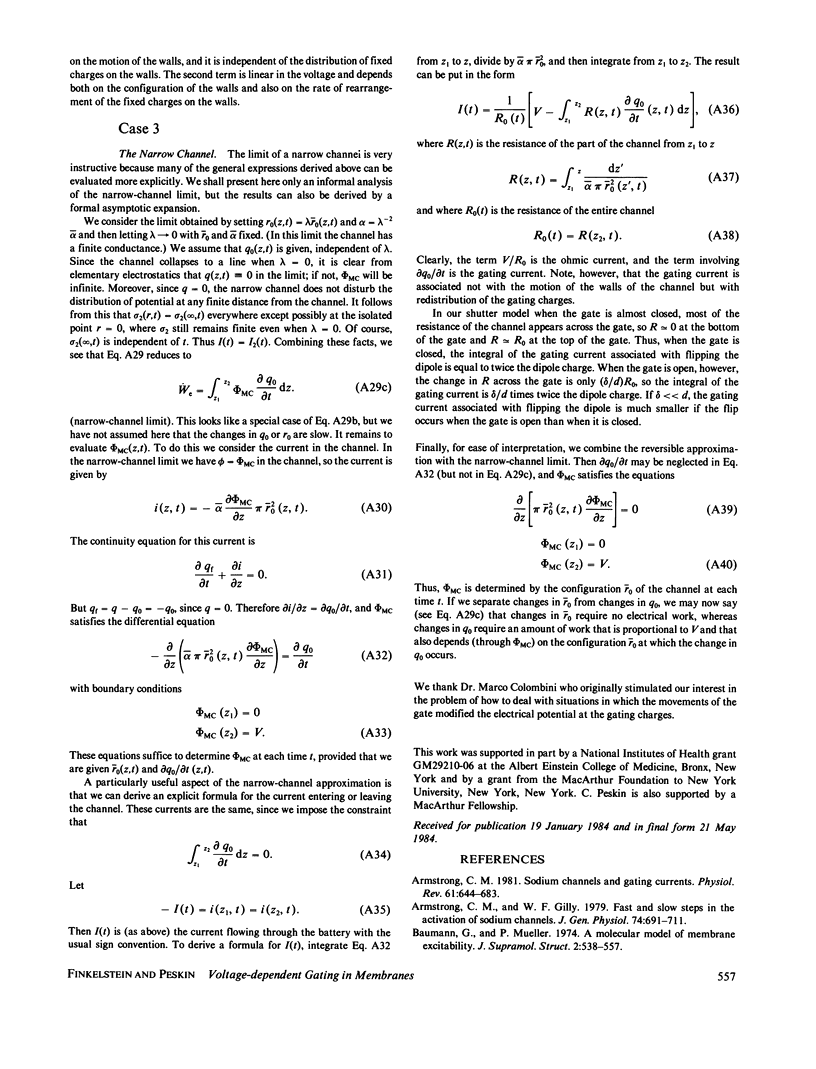
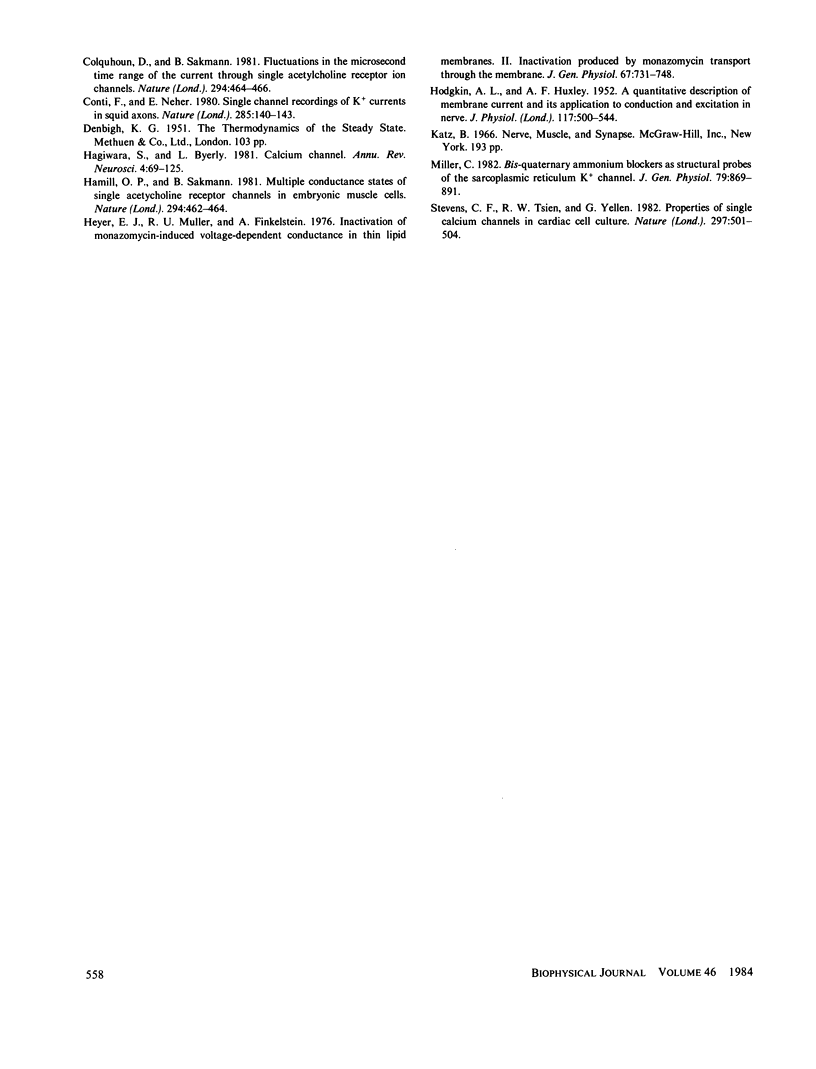
Selected References
These references are in PubMed. This may not be the complete list of references from this article.
- Armstrong C. M., Gilly W. F. Fast and slow steps in the activation of sodium channels. J Gen Physiol. 1979 Dec;74(6):691–711. doi: 10.1085/jgp.74.6.691. [DOI] [PMC free article] [PubMed] [Google Scholar]
- Armstrong C. M. Sodium channels and gating currents. Physiol Rev. 1981 Jul;61(3):644–683. doi: 10.1152/physrev.1981.61.3.644. [DOI] [PubMed] [Google Scholar]
- Baumann G., Mueller P. A molecular model of membrane excitability. J Supramol Struct. 1974;2(5-6):538–557. doi: 10.1002/jss.400020504. [DOI] [PubMed] [Google Scholar]
- Colquhoun D., Sakmann B. Fluctuations in the microsecond time range of the current through single acetylcholine receptor ion channels. Nature. 1981 Dec 3;294(5840):464–466. doi: 10.1038/294464a0. [DOI] [PubMed] [Google Scholar]
- Conti F., Neher E. Single channel recordings of K+ currents in squid axons. Nature. 1980 May 15;285(5761):140–143. doi: 10.1038/285140a0. [DOI] [PubMed] [Google Scholar]
- HODGKIN A. L., HUXLEY A. F. A quantitative description of membrane current and its application to conduction and excitation in nerve. J Physiol. 1952 Aug;117(4):500–544. doi: 10.1113/jphysiol.1952.sp004764. [DOI] [PMC free article] [PubMed] [Google Scholar]
- Hagiwara S., Byerly L. Calcium channel. Annu Rev Neurosci. 1981;4:69–125. doi: 10.1146/annurev.ne.04.030181.000441. [DOI] [PubMed] [Google Scholar]
- Hamill O. P., Sakmann B. Multiple conductance states of single acetylcholine receptor channels in embryonic muscle cells. Nature. 1981 Dec 3;294(5840):462–464. doi: 10.1038/294462a0. [DOI] [PubMed] [Google Scholar]
- Heyer R. J., Muller R. U., Finkelstein A. Inactivation of monazomycin-induced voltage-dependent conductance in thin lipid membranes. II. Inactivation produced by monazomycin transport through the membrane. J Gen Physiol. 1976 Jun;67(6):731–748. doi: 10.1085/jgp.67.6.731. [DOI] [PMC free article] [PubMed] [Google Scholar]
- Miller C. Bis-quaternary ammonium blockers as structural probes of the sarcoplasmic reticulum K+ channel. J Gen Physiol. 1982 May;79(5):869–891. doi: 10.1085/jgp.79.5.869. [DOI] [PMC free article] [PubMed] [Google Scholar]
- Reuter H., Stevens C. F., Tsien R. W., Yellen G. Properties of single calcium channels in cardiac cell culture. Nature. 1982 Jun 10;297(5866):501–504. doi: 10.1038/297501a0. [DOI] [PubMed] [Google Scholar]