Abstract
Agrobacterium radiobacter NCIB 11883 was grown in lactose-limited continuous culture at a dilution rate of 0.045/h. Washed cells transported [14C]lactose and [methyl-14C]beta-D-thiogalactoside, a nonmetabolisable analog of lactose, at similar rates and with similar affinities (Km for transport, less than 1 microM). Transport was inhibited to various extents by the uncoupling agent carbonyl cyanide p-trifluoromethoxyphenylhydrazone, by unlabeled beta-galactosides and D-galactose, and by osmotic shock. The accumulation ratio for methyl-beta-D-thiogalactoside was greater than or equal to 4,100. An abundant protein (molecular weight, 41,000) was purified from osmotic-shock fluid and shown by equilibrium dialysis to bind lactose and methyl-beta-D-thiogalactoside, the former with very high affinity (binding constant, 0.14 microM). The N-terminal amino acid sequence of this lactose-binding protein exhibited some homology with several other sugar-binding proteins from bacteria. Antiserum raised against the lactose-binding protein did not cross-react with two glucose-binding proteins from A. radiobacter or with extracts of other bacteria grown under lactose limitation. Lactose transport and beta-galactosidase were induced in batch cultures by lactose, melibiose [O-alpha-D-galactoside-(1----6)alpha-D-glucose], and isopropyl-beta-D-thiogalactoside and were subject to catabolite repression by glucose, galactose, and succinate which was not alleviated by cyclic AMP. We conclude that lactose is transported into A. radiobacter via a binding protein-dependent active transport system (in contrast to the H+ symport and phosphotransferase systems found in other bacteria) and that the expression of this transport system is closely linked to that of beta-galactosidase.
Full text
PDF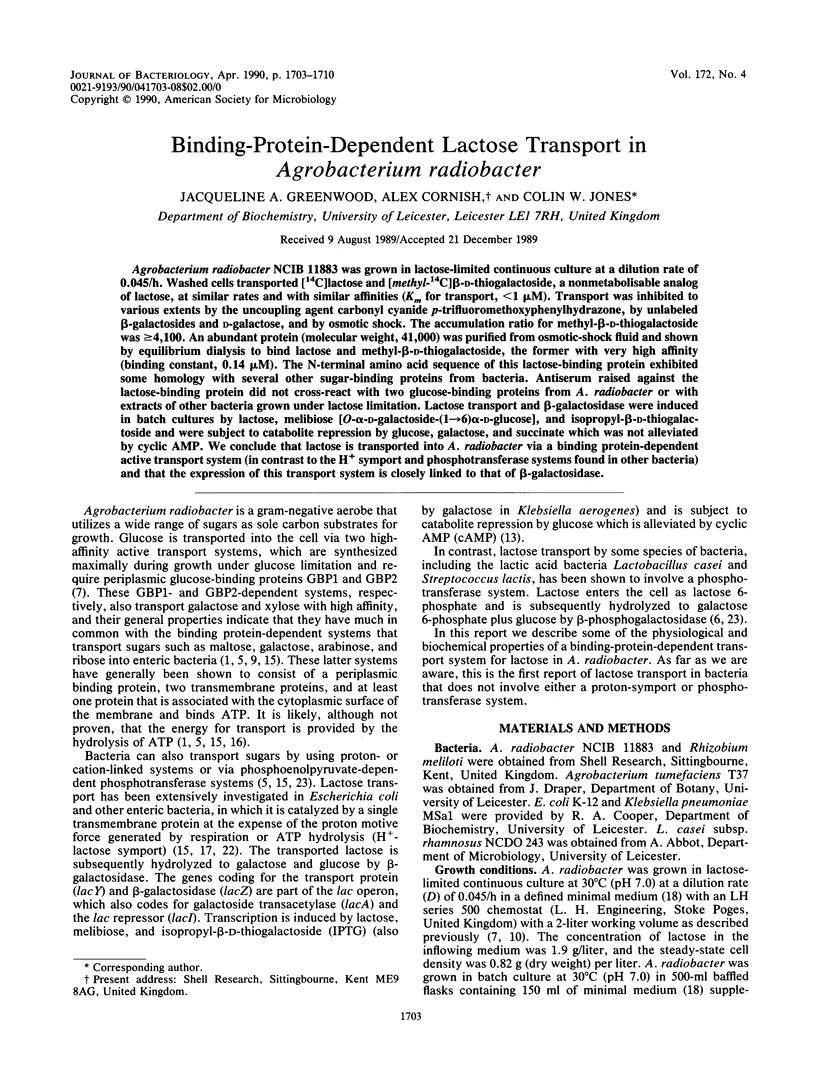
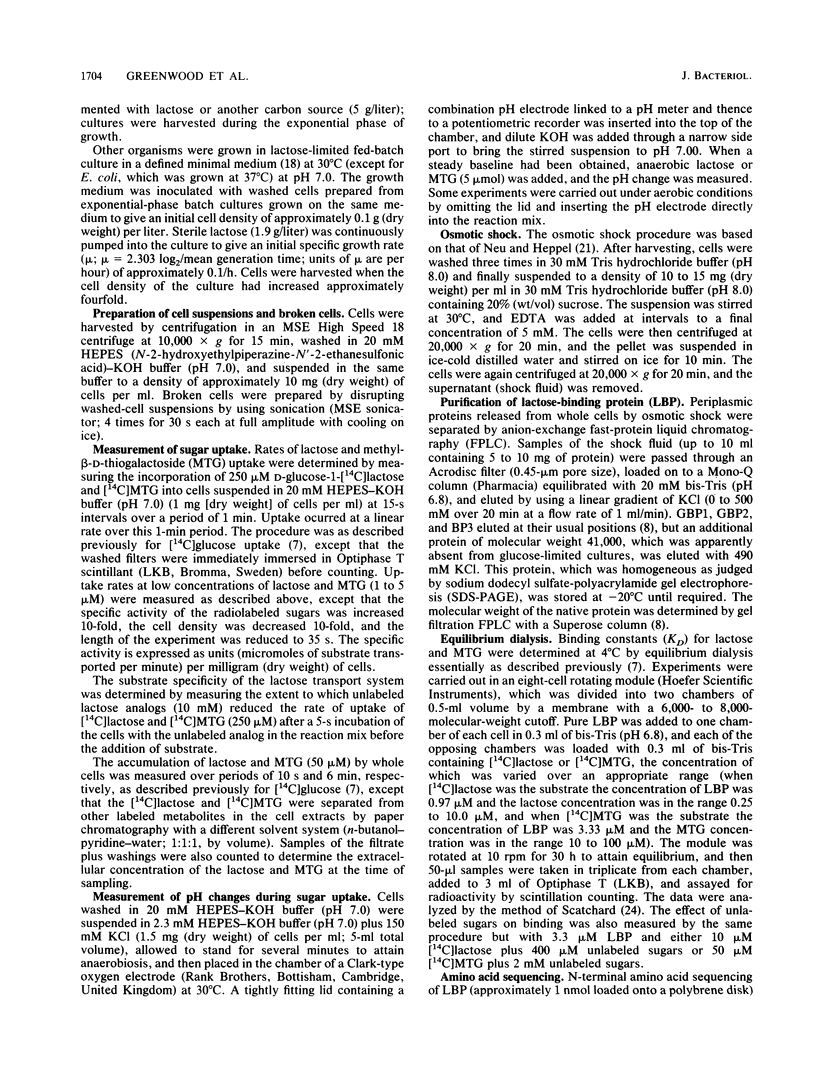
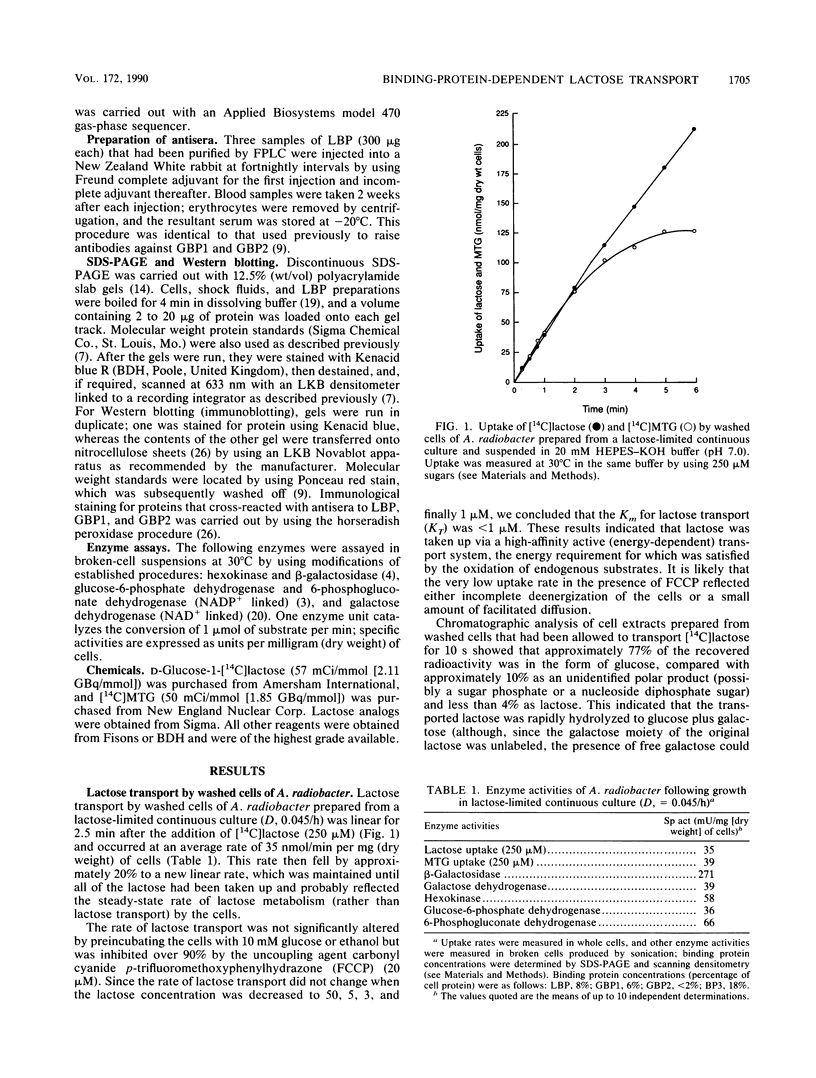
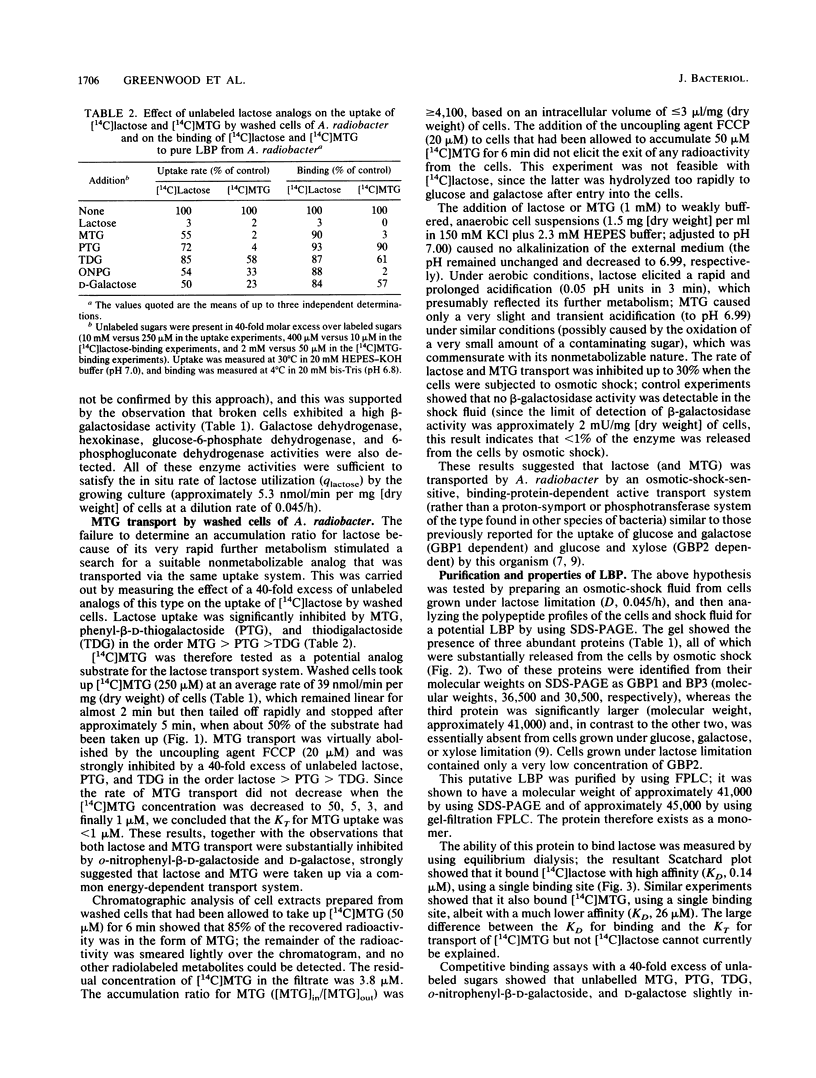
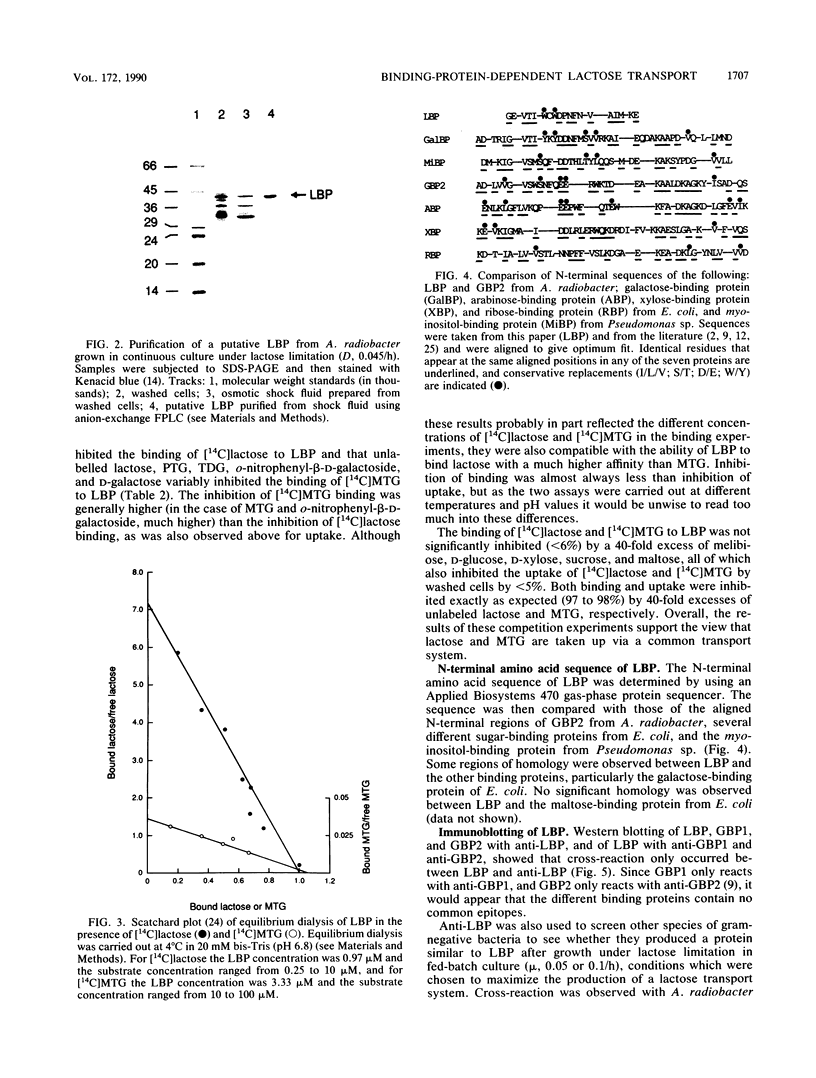
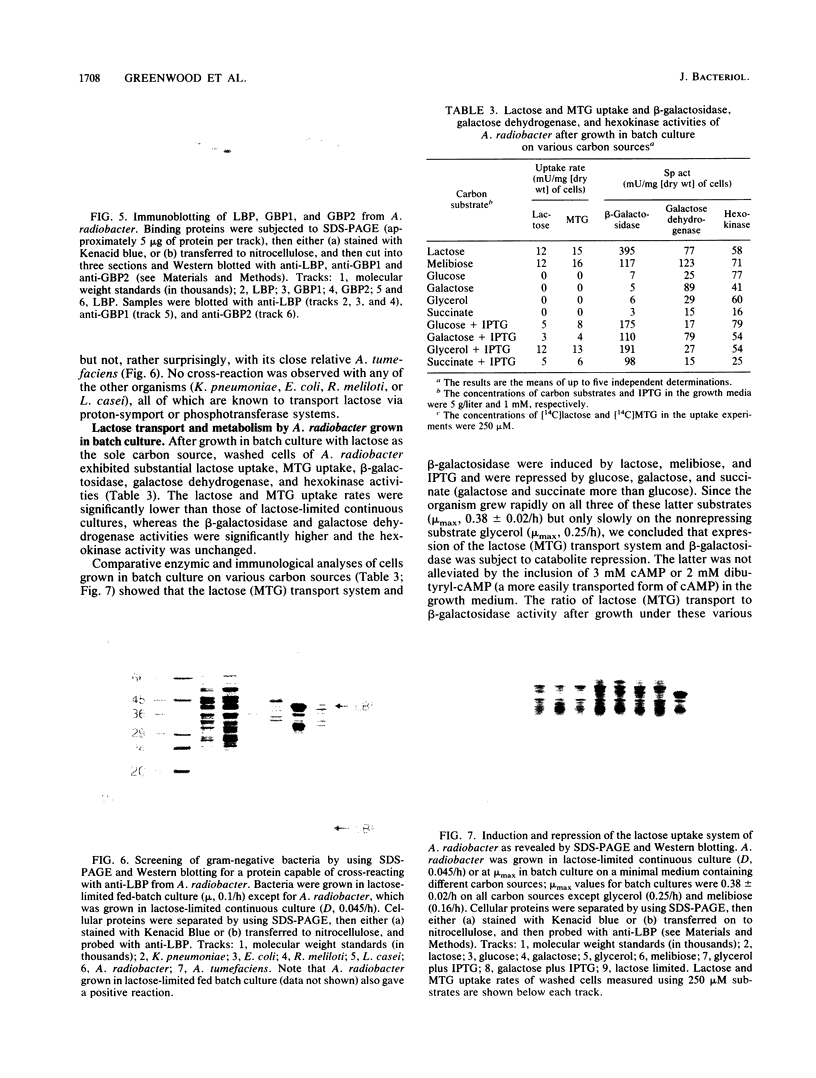
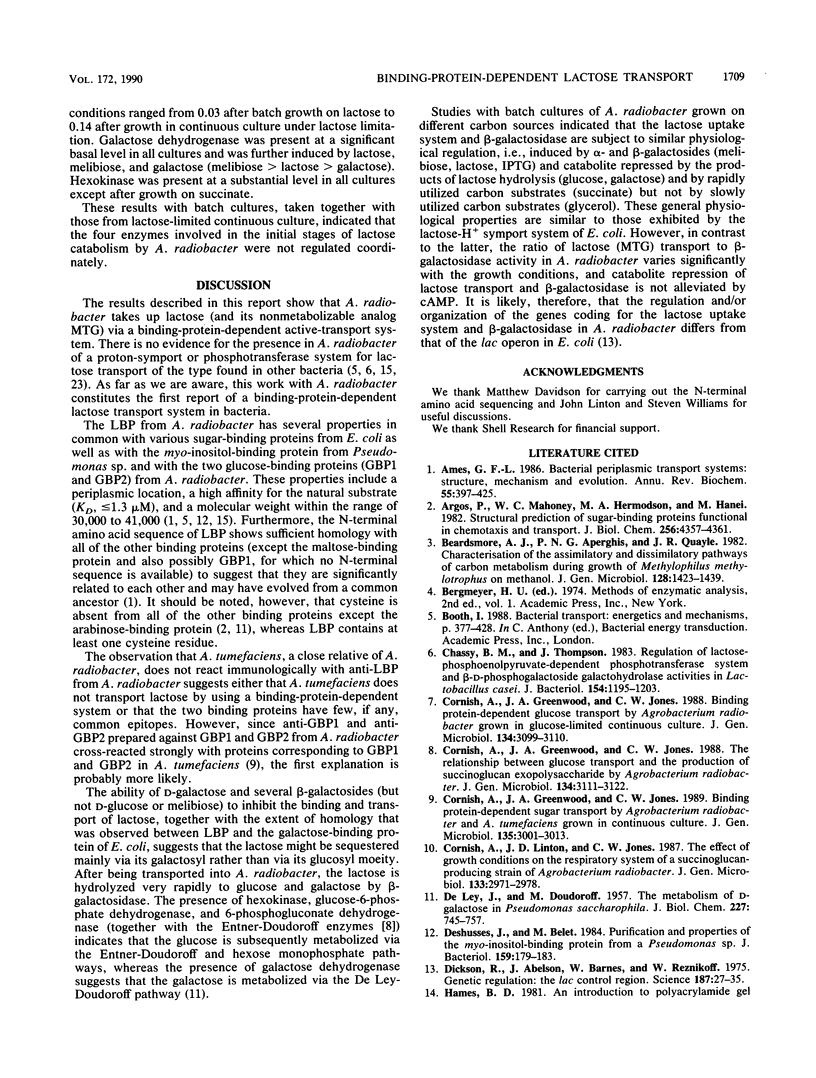
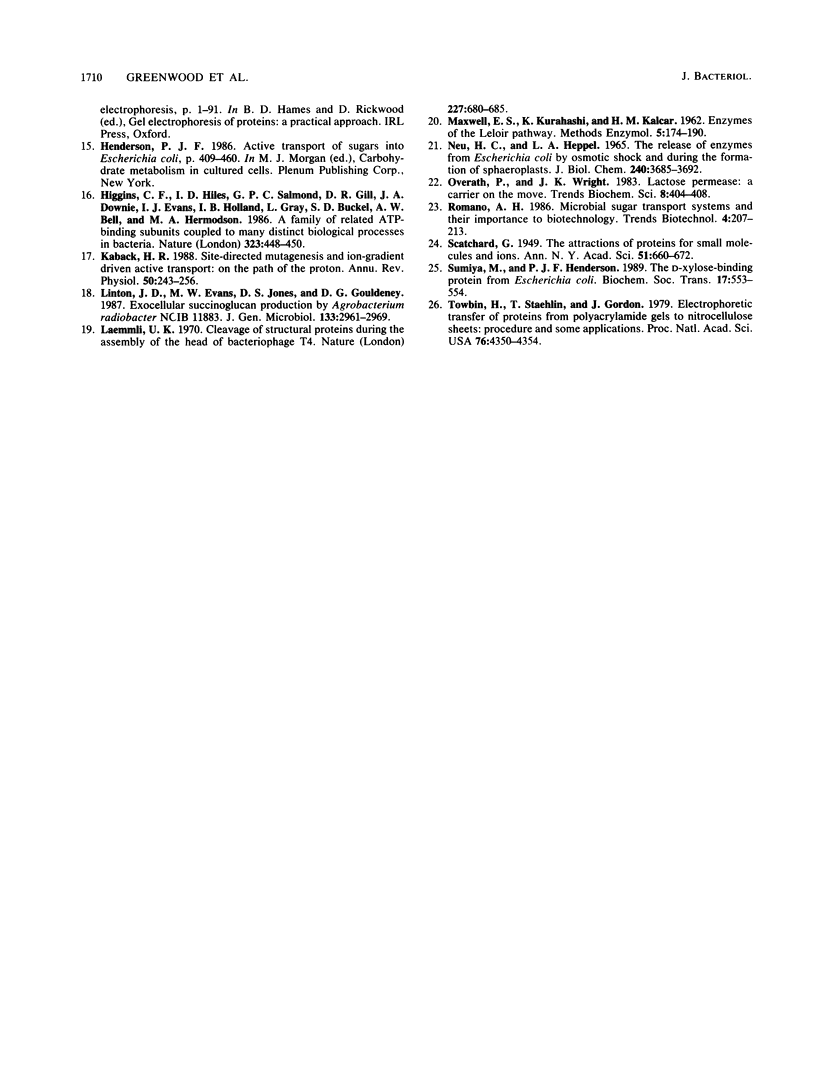
Images in this article
Selected References
These references are in PubMed. This may not be the complete list of references from this article.
- Ames G. F. Bacterial periplasmic transport systems: structure, mechanism, and evolution. Annu Rev Biochem. 1986;55:397–425. doi: 10.1146/annurev.bi.55.070186.002145. [DOI] [PubMed] [Google Scholar]
- Argos P., Mahoney W. C., Hermodson M. A., Hanei M. Structural prediction of sugar-binding proteins functional in chemotaxis and transport. J Biol Chem. 1981 May 10;256(9):4357–4361. [PubMed] [Google Scholar]
- Chassy B. M., Thompson J. Regulation of lactose-phosphoenolpyruvate-dependent phosphotransferase system and beta-D-phosphogalactoside galactohydrolase activities in Lactobacillus casei. J Bacteriol. 1983 Jun;154(3):1195–1203. doi: 10.1128/jb.154.3.1195-1203.1983. [DOI] [PMC free article] [PubMed] [Google Scholar]
- Cornish A., Greenwood J. A., Jones C. W. Binding-protein-dependent glucose transport by Agrobacterium radiobacter grown in glucose-limited continuous culture. J Gen Microbiol. 1988 Dec;134(12):3099–3110. doi: 10.1099/00221287-134-12-3099. [DOI] [PubMed] [Google Scholar]
- Cornish A., Greenwood J. A., Jones C. W. Binding-protein-dependent sugar transport by Agrobacterium radiobacter and A. tumefaciens grown in continuous culture. J Gen Microbiol. 1989 Nov;135(11):3001–3013. doi: 10.1099/00221287-135-11-3001. [DOI] [PubMed] [Google Scholar]
- Cornish A., Greenwood J. A., Jones C. W. The relationship between glucose transport and the production of succinoglucan exopolysaccharide by Agrobacterium radiobacter. J Gen Microbiol. 1988 Dec;134(12):3111–3122. doi: 10.1099/00221287-134-12-3111. [DOI] [PubMed] [Google Scholar]
- DE LEY J., DOUDOROFF M. The metabolism of D-galactose in Pseudomonas saccharophila. J Biol Chem. 1957 Aug;227(2):745–757. [PubMed] [Google Scholar]
- Deshusses J., Belet M. Purification and properties of the myo-inositol-binding protein from a Pseudomonas sp. J Bacteriol. 1984 Jul;159(1):179–183. doi: 10.1128/jb.159.1.179-183.1984. [DOI] [PMC free article] [PubMed] [Google Scholar]
- Dickson R. C., Abelson J., Barnes W. M., Reznikoff W. S. Genetic regulation: the Lac control region. Science. 1975 Jan 10;187(4171):27–35. doi: 10.1126/science.1088926. [DOI] [PubMed] [Google Scholar]
- Higgins C. F., Hiles I. D., Salmond G. P., Gill D. R., Downie J. A., Evans I. J., Holland I. B., Gray L., Buckel S. D., Bell A. W. A family of related ATP-binding subunits coupled to many distinct biological processes in bacteria. Nature. 1986 Oct 2;323(6087):448–450. doi: 10.1038/323448a0. [DOI] [PubMed] [Google Scholar]
- Kaback H. R. Site-directed mutagenesis and ion-gradient driven active transport: on the path of the proton. Annu Rev Physiol. 1988;50:243–256. doi: 10.1146/annurev.ph.50.030188.001331. [DOI] [PubMed] [Google Scholar]
- Laemmli U. K. Cleavage of structural proteins during the assembly of the head of bacteriophage T4. Nature. 1970 Aug 15;227(5259):680–685. doi: 10.1038/227680a0. [DOI] [PubMed] [Google Scholar]
- Neu H. C., Heppel L. A. The release of enzymes from Escherichia coli by osmotic shock and during the formation of spheroplasts. J Biol Chem. 1965 Sep;240(9):3685–3692. [PubMed] [Google Scholar]
- Towbin H., Staehelin T., Gordon J. Electrophoretic transfer of proteins from polyacrylamide gels to nitrocellulose sheets: procedure and some applications. Proc Natl Acad Sci U S A. 1979 Sep;76(9):4350–4354. doi: 10.1073/pnas.76.9.4350. [DOI] [PMC free article] [PubMed] [Google Scholar]