Abstract
Stable mammalian cell lines harboring a synthetic bovine opsin gene have been derived from the suspension-adapted HEK293 cell line. The opsin gene is under the control of the immediate-early cytomegalovirus promoter/enhancer in an expression vector that also contains a selectable marker (Neo) governed by a relatively weak promoter. The cell lines expressing the opsin gene at high levels are selected by growth in the presence of high concentrations of the antibiotic geneticin. Under the conditions used for cell growth in suspension, opsin is produced at saturated culture levels of more than 2 mg/liter. After reconstitution with 11-cis-retinal, rhodopsin is purified to homogeneity in a single step by immunoaffinity column chromatography. Rhodopsin thus prepared (> 90% recovery at concentrations of up to 15 microM) is indistinguishable from rhodopsin purified from bovine rod outer segments by the following criteria: (i) UV/Vis absorption spectra in the dark and after photobleaching and the rate of metarhodopsin II decay, (ii) initial rates of transducin activation, and (iii) the rate of phosphorylation by rhodopsin kinase. Although mammalian cell opsin migrates slower than rod outer segment opsin on SDS/polyacrylamide gels, presumably due to a different N-glycosylation pattern, their mobilities after deglycosylation are identical. This method has enabled the preparation of several site-specific mutants of bovine opsin in comparable amounts.
Full text
PDF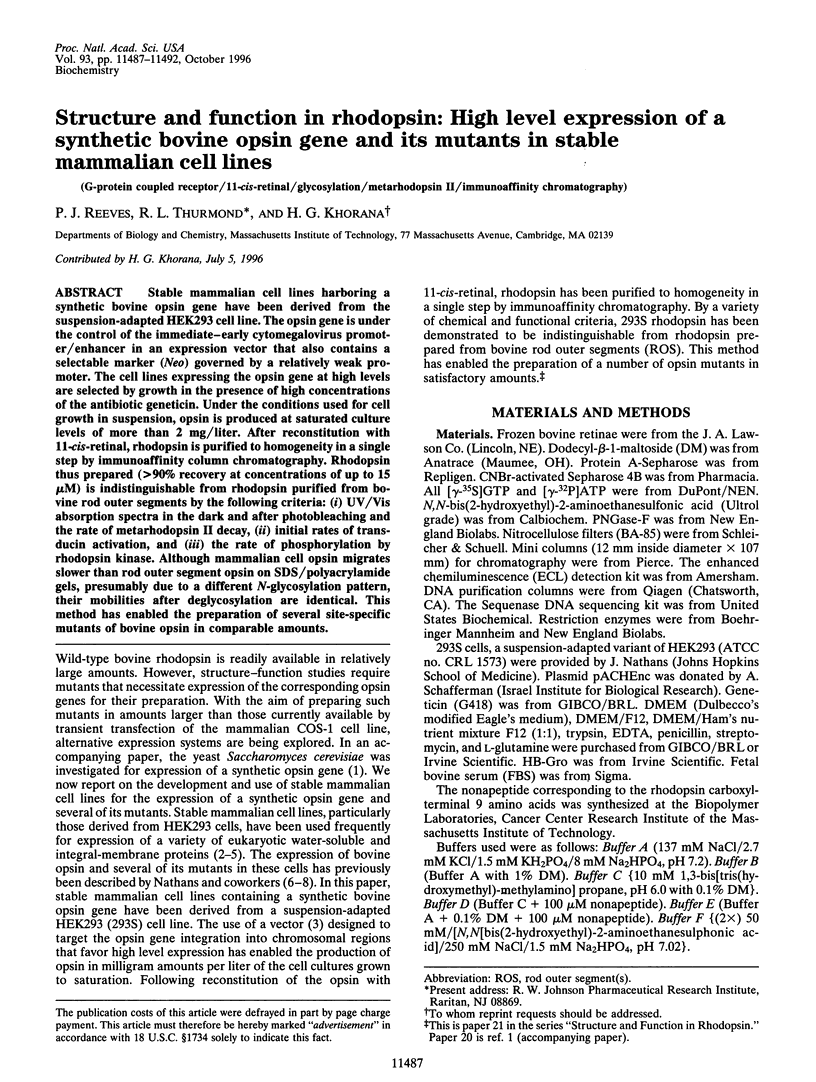
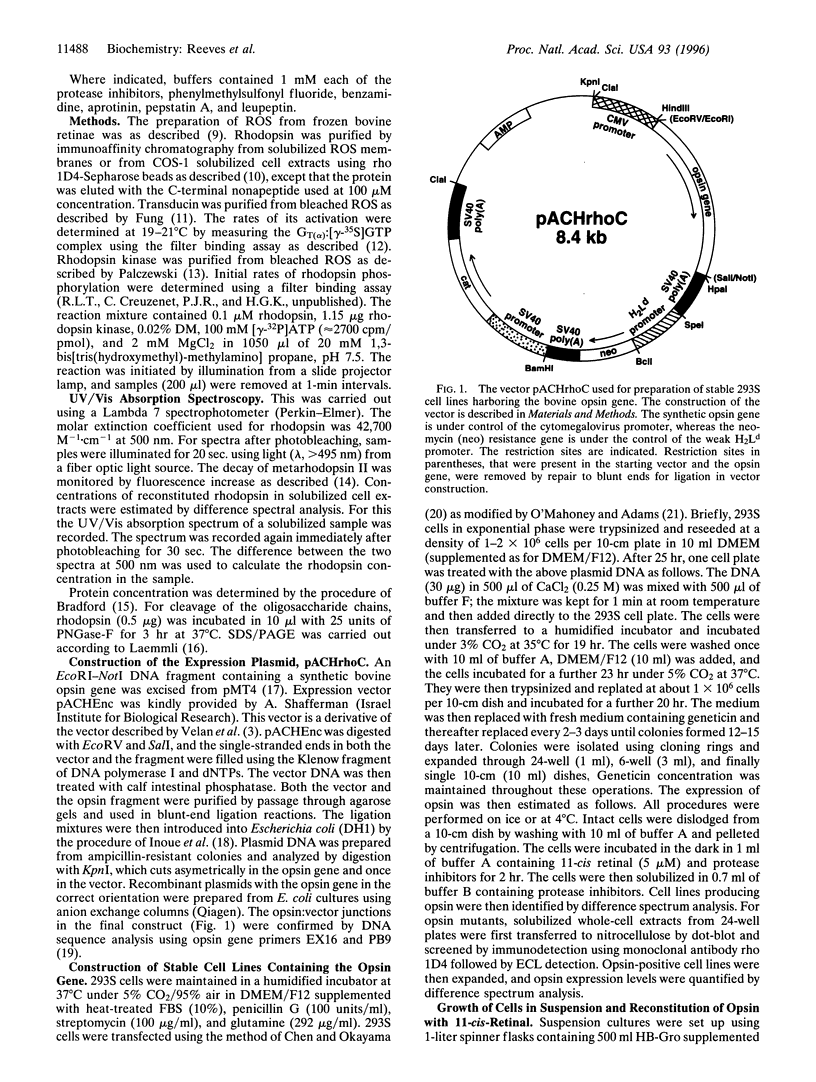
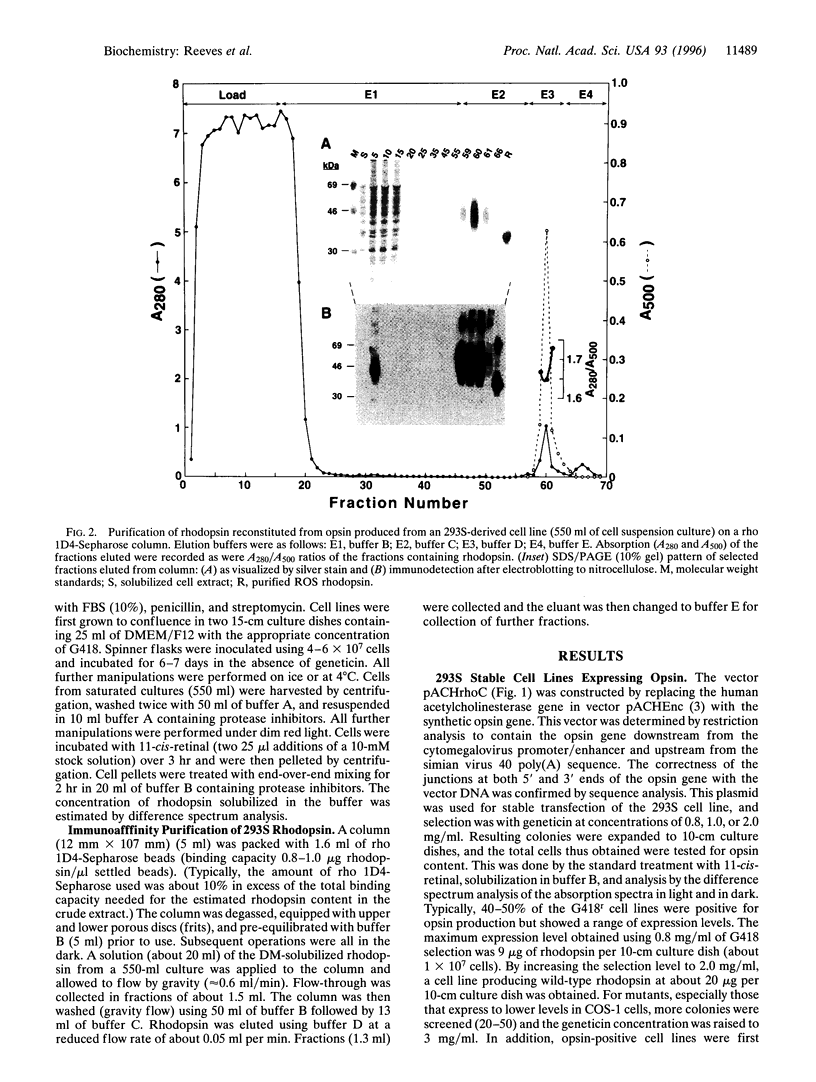
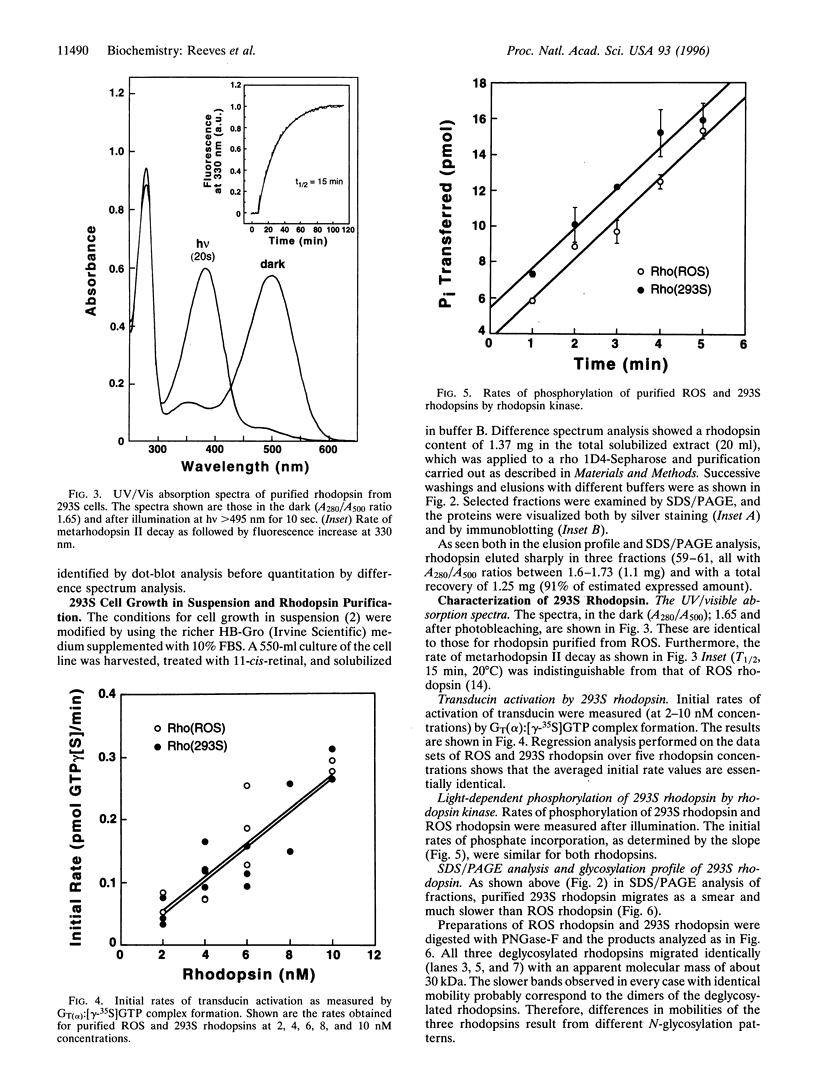
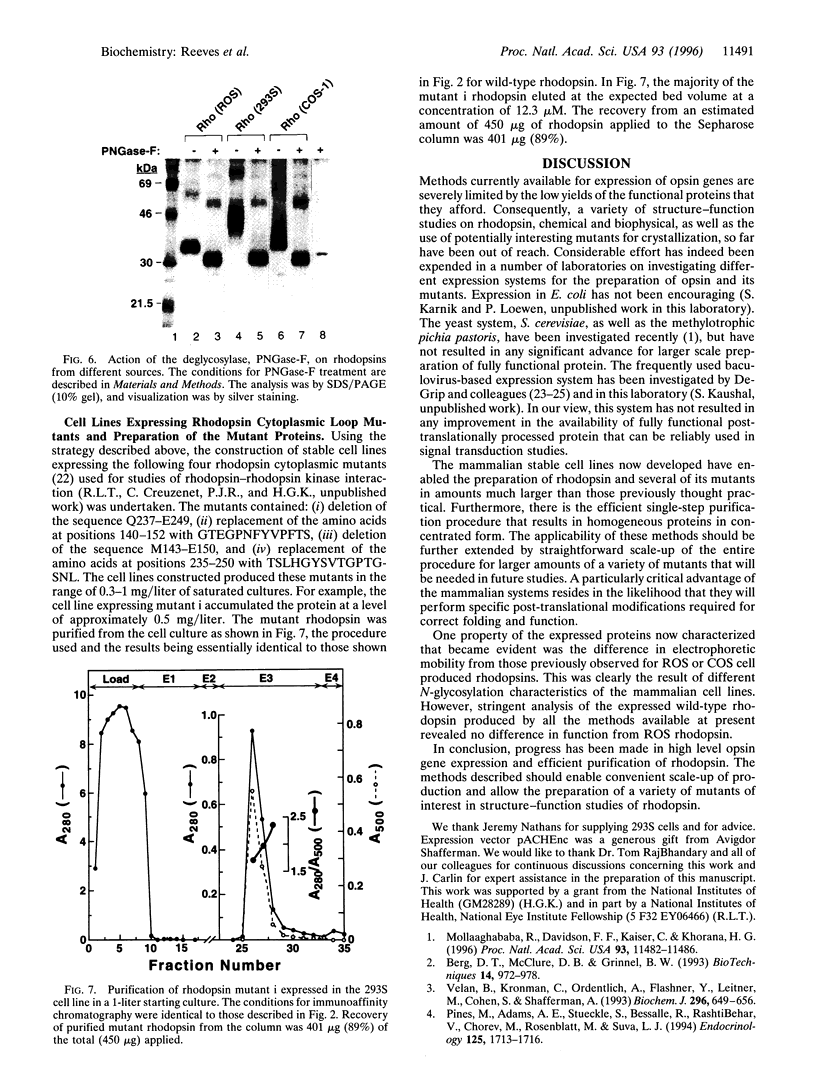
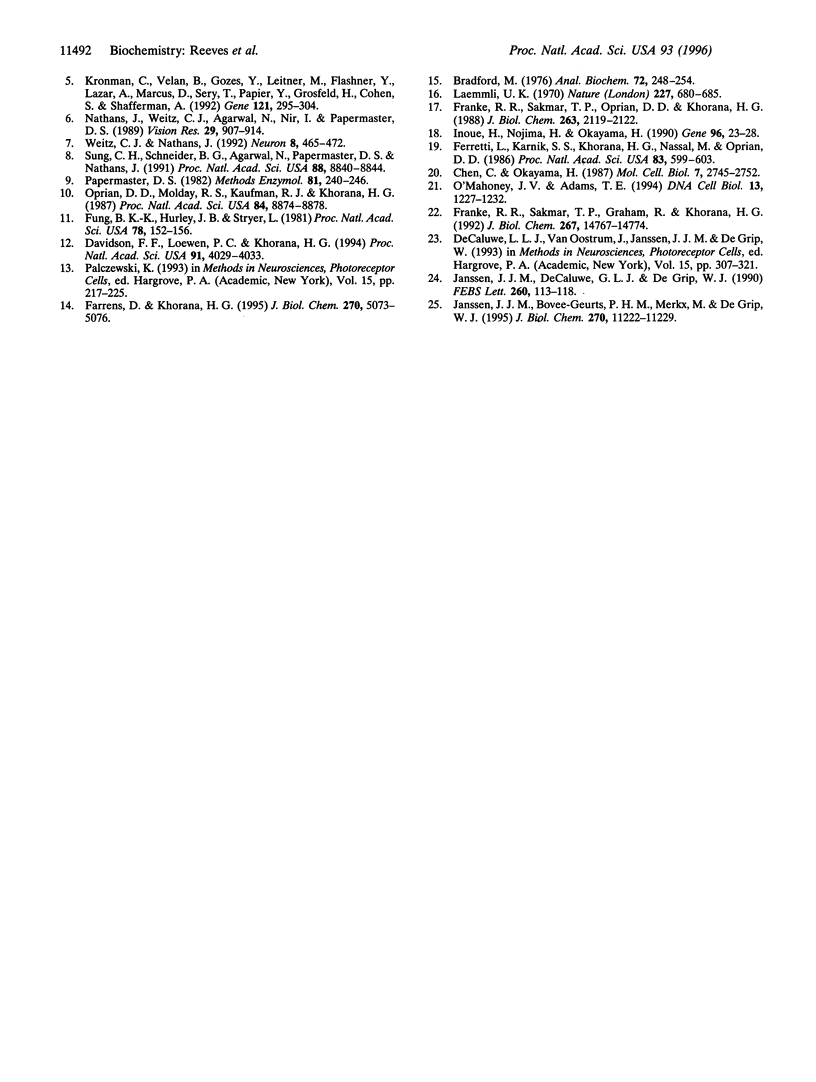
Images in this article
Selected References
These references are in PubMed. This may not be the complete list of references from this article.
- Berg D. T., McClure D. B., Grinnell B. W. High-level expression of secreted proteins from cells adapted to serum-free suspension culture. Biotechniques. 1993 Jun;14(6):972–978. [PubMed] [Google Scholar]
- Bradford M. M. A rapid and sensitive method for the quantitation of microgram quantities of protein utilizing the principle of protein-dye binding. Anal Biochem. 1976 May 7;72:248–254. doi: 10.1016/0003-2697(76)90527-3. [DOI] [PubMed] [Google Scholar]
- Chen C., Okayama H. High-efficiency transformation of mammalian cells by plasmid DNA. Mol Cell Biol. 1987 Aug;7(8):2745–2752. doi: 10.1128/mcb.7.8.2745. [DOI] [PMC free article] [PubMed] [Google Scholar]
- Davidson F. F., Loewen P. C., Khorana H. G. Structure and function in rhodopsin: replacement by alanine of cysteine residues 110 and 187, components of a conserved disulfide bond in rhodopsin, affects the light-activated metarhodopsin II state. Proc Natl Acad Sci U S A. 1994 Apr 26;91(9):4029–4033. doi: 10.1073/pnas.91.9.4029. [DOI] [PMC free article] [PubMed] [Google Scholar]
- Farrens D. L., Khorana H. G. Structure and function in rhodopsin. Measurement of the rate of metarhodopsin II decay by fluorescence spectroscopy. J Biol Chem. 1995 Mar 10;270(10):5073–5076. doi: 10.1074/jbc.270.10.5073. [DOI] [PubMed] [Google Scholar]
- Ferretti L., Karnik S. S., Khorana H. G., Nassal M., Oprian D. D. Total synthesis of a gene for bovine rhodopsin. Proc Natl Acad Sci U S A. 1986 Feb;83(3):599–603. doi: 10.1073/pnas.83.3.599. [DOI] [PMC free article] [PubMed] [Google Scholar]
- Franke R. R., Sakmar T. P., Graham R. M., Khorana H. G. Structure and function in rhodopsin. Studies of the interaction between the rhodopsin cytoplasmic domain and transducin. J Biol Chem. 1992 Jul 25;267(21):14767–14774. [PubMed] [Google Scholar]
- Franke R. R., Sakmar T. P., Oprian D. D., Khorana H. G. A single amino acid substitution in rhodopsin (lysine 248----leucine) prevents activation of transducin. J Biol Chem. 1988 Feb 15;263(5):2119–2122. [PubMed] [Google Scholar]
- Fung B. K., Hurley J. B., Stryer L. Flow of information in the light-triggered cyclic nucleotide cascade of vision. Proc Natl Acad Sci U S A. 1981 Jan;78(1):152–156. doi: 10.1073/pnas.78.1.152. [DOI] [PMC free article] [PubMed] [Google Scholar]
- Inoue H., Nojima H., Okayama H. High efficiency transformation of Escherichia coli with plasmids. Gene. 1990 Nov 30;96(1):23–28. doi: 10.1016/0378-1119(90)90336-p. [DOI] [PubMed] [Google Scholar]
- Janssen J. J., Bovee-Geurts P. H., Merkx M., DeGrip W. J. Histidine tagging both allows convenient single-step purification of bovine rhodopsin and exerts ionic strength-dependent effects on its photochemistry. J Biol Chem. 1995 May 12;270(19):11222–11229. doi: 10.1074/jbc.270.19.11222. [DOI] [PubMed] [Google Scholar]
- Janssen J. J., De Caluwé G. L., De Grip W. J. Asp83, Glu113 and Glu134 are not specifically involved in Schiff base protonation or wavelength regulation in bovine rhodopsin. FEBS Lett. 1990 Jan 15;260(1):113–118. doi: 10.1016/0014-5793(90)80080-3. [DOI] [PubMed] [Google Scholar]
- Kronman C., Velan B., Gozes Y., Leitner M., Flashner Y., Lazar A., Marcus D., Sery T., Papier Y., Grosfeld H. Production and secretion of high levels of recombinant human acetylcholinesterase in cultured cell lines: microheterogeneity of the catalytic subunit. Gene. 1992 Nov 16;121(2):295–304. doi: 10.1016/0378-1119(92)90134-b. [DOI] [PubMed] [Google Scholar]
- Laemmli U. K. Cleavage of structural proteins during the assembly of the head of bacteriophage T4. Nature. 1970 Aug 15;227(5259):680–685. doi: 10.1038/227680a0. [DOI] [PubMed] [Google Scholar]
- Mollaaghababa R., Davidson F. F., Kaiser C., Khorana H. G. Structure and function in rhodopsin: expression of functional mammalian opsin in Saccharomyces cerevisiae. Proc Natl Acad Sci U S A. 1996 Oct 15;93(21):11482–11486. doi: 10.1073/pnas.93.21.11482. [DOI] [PMC free article] [PubMed] [Google Scholar]
- Nathans J., Weitz C. J., Agarwal N., Nir I., Papermaster D. S. Production of bovine rhodopsin by mammalian cell lines expressing cloned cDNA: spectrophotometry and subcellular localization. Vision Res. 1989;29(8):907–914. doi: 10.1016/0042-6989(89)90105-3. [DOI] [PubMed] [Google Scholar]
- O'Mahoney J. V., Adams T. E. Optimization of experimental variables influencing reporter gene expression in hepatoma cells following calcium phosphate transfection. DNA Cell Biol. 1994 Dec;13(12):1227–1232. doi: 10.1089/dna.1994.13.1227. [DOI] [PubMed] [Google Scholar]
- Oprian D. D., Molday R. S., Kaufman R. J., Khorana H. G. Expression of a synthetic bovine rhodopsin gene in monkey kidney cells. Proc Natl Acad Sci U S A. 1987 Dec;84(24):8874–8878. doi: 10.1073/pnas.84.24.8874. [DOI] [PMC free article] [PubMed] [Google Scholar]
- Papermaster D. S. Preparation of antibodies to rhodopsin and the large protein of rod outer segments. Methods Enzymol. 1982;81:240–246. doi: 10.1016/s0076-6879(82)81037-9. [DOI] [PubMed] [Google Scholar]
- Pines M., Adams A. E., Stueckle S., Bessalle R., Rashti-Behar V., Chorev M., Rosenblatt M., Suva L. J. Generation and characterization of human kidney cell lines stably expressing recombinant human PTH/PTHrP receptor: lack of interaction with a C-terminal human PTH peptide. Endocrinology. 1994 Oct;135(4):1713–1716. doi: 10.1210/endo.135.4.7925136. [DOI] [PubMed] [Google Scholar]
- Sung C. H., Schneider B. G., Agarwal N., Papermaster D. S., Nathans J. Functional heterogeneity of mutant rhodopsins responsible for autosomal dominant retinitis pigmentosa. Proc Natl Acad Sci U S A. 1991 Oct 1;88(19):8840–8844. doi: 10.1073/pnas.88.19.8840. [DOI] [PMC free article] [PubMed] [Google Scholar]
- Velan B., Kronman C., Ordentlich A., Flashner Y., Leitner M., Cohen S., Shafferman A. N-glycosylation of human acetylcholinesterase: effects on activity, stability and biosynthesis. Biochem J. 1993 Dec 15;296(Pt 3):649–656. doi: 10.1042/bj2960649. [DOI] [PMC free article] [PubMed] [Google Scholar]
- Weitz C. J., Nathans J. Histidine residues regulate the transition of photoexcited rhodopsin to its active conformation, metarhodopsin II. Neuron. 1992 Mar;8(3):465–472. doi: 10.1016/0896-6273(92)90274-h. [DOI] [PubMed] [Google Scholar]